Fish Decline, Caused by Global Warming, May Leave Many Hungry in the Amazon
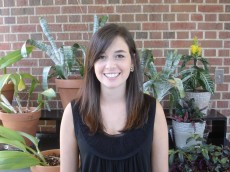
Elizabeth Ross
Download the PDF
Abstract
The world loves fish. In fact, global consumption of fish and fishery products has risen by 240% since 1960 and projections raise the possibility that this year could show another 50% jump (1). Yet this surge in our appetite for fish is happening against a backdrop of dramatic climate change that’s reducing the survival rates of young fish. As the temperature of our oceans rise, the fish- and the indigenous peoples of the developing world who depend on them as a critical source of protein- are feeling it. Whether fish or human, when life is fragile there is no such thing as a small change.
Introduction
Region
The world loves fish. It’s a favorite food for many around the globe, and for nearly a billion people, most of them in developing countries, fish is a critical source of protein. And our worldwide hunger for fish is growing. Fast. Global consumption of fish and fishery products has risen by 240 percent since 1960. Projections show the current year to bump that growth by another 34 to 50 percent (1).
This surge in our appetite for fish is happening against a backdrop of dramatic climate change. The temperature of our water supply is rising. During the past 40 years, water temperatures in the German Bight, for example, increased by 1.13°C. Models predict further sea surface temperature increments for the next 90 to 100 years, by about 1.6° to 3.0°C in the northern and even by 3.0° to 3.9°C in the shallower southern North Sea (2). The patterns of marine species richness, notably for fish and invertebrates, are strongly related to environmental factors (3), causing this increase in temperature to have serious implications on the world’s fish populations.
Recent studies have found that fish are moving towards the poles, pteropods and coral reefs are responding negatively to the increasing atmospheric carbon dioxide concentrations and rising water temperatures are modifying plankton ecosystems in a way that reduces the survival of young fish. These negative responses to warming waters not only have big implications for fish, they have big implications for the 1/6th of our planet that currently depend on fish for its primary source of protein (1). Many may be forced to look at other sources for their protein intake.
Fish Decline
Shift in Habitat
The productivity of marine fisheries is a fragile business. Ocean conditions including water temperature, currents and costal upwellings, all have an impact (4). Because they are ectotherms, the body temperatures of fish vary with the temperature of the surrounding environment, making them extremely responsive to any sudden change in water temperature. Recent studies have shown fish are responding to the warming waters by shifting their distributions towards higher latitudes and deeper waters (4). This results in high invasion intensity and overall increase of species richness in high latitude (>40°N and >30°S) regions (3). Twenty-one of thirty-six species of demersal (bottom-living) fish in the North Sea have shown this distributional response to climatic warming. The North Sea has warmed by an average of 1.05°C between 1977 and 2001 (5). Species such as the Atlantic cod (Gadus morhua), the common sole (Solea solea), the scaldfish (Arnoglossus laterna) and the snake-blenny (Lumpenus lampretaeformis)
have shifted from their mean latitude distances ranging from48 to 403 km (5). Most of the 90 species studied moved northward and shifted their depth. This change is expected due to the positive correlation between the North Sea depths and latitude (5). Now, huge populations of fish are seeking and conquering new territories, possibly affecting the delicate food chains of both their new and former homes.
Ocean Acidification
Today’s ocean is saturated with calcium carbonate. But increasing atmospheric carbon dioxide concentrations due to global climate change are reducing ocean pH and carbonate ion concentrations, and thus the level of calcium carbonate saturation (6). Approximately 25% of the CO2 emitted from all anthropogenic sources currently enter sthe ocean where it reacts with water to produce carbonic acid (7). This acid dissociates to form bicarbonate ions and protons, which in turn react with carbonate ions to produce more bicarbonate ions, reducing the availability of carbonate to biological systems (7). The decreasing carbonate-ion concentrates reduce the rate of calcification of marine organisms such as reef-building corals and pteropods. This is no small worry.
Experimental studies have shown that a doubling of pre-industrial [CO2]atm to 560 ppm decreases coral calcification and growth by up to 40% through the inhibition of aragonite formation (the principal crystalline form of calcium carbonate deposited in coral skeletons) as carbonate-ion concentrations decrease (8). Reef-building corals are expected to exhibit several responses to reduced calcification, all of which have deleterious consequences for reef ecosystems. The most direct response is a decreased linear extension rate and skeletal density of the coral colonies, leading to both erosion and a greater risk of storm damage. If rates of erosion exceed calcification, the structural complexity of coral reefs will diminish, and thus reduce habitat quality and diversity (7).
Another possible response is an energy diversion effect in which the coral reefs divert energy from other systems to compensate for the reduced skeletal growth and density that occurs under reduced carbonate saturation (7). A realistic side effect of this would be the diversion of resources from other essential processes such as reproduction, ultimately reducing the potential for re-colonization after natural disturbances (7). Unfortunately, modern genotypes and phenotypes of corals do not appear to have the capacity to adapt fast enough to the sudden environmental change.
Coral reefs are among the more biologically diverse and economically important ecosystems on the planet, providing ecosystem services to hundreds of thousands of marine organisms that inhabit the reefs. Yet the dangers of a less productive coral system go way beyond the sea itself. The reefs, and the life they inspire, support and protection are vital to human societies and industries through fisheries, coastal protection, building materials, new biochemical compounds, and tourism (9).
Changing water temperatures have affected another building block of the sea. Pteropods, small floating snails, which are eaten by various species of fish, may be unable to maintain their shells in water that is becoming under-saturated with aragonite (6), a compound also influenced by CO2. Sediment trap studies have found that empty pteropod shells exhibited pitting and partial dissolution as soon as they fall below the aragonite saturation horizon (10). New experimental evidence suggests that even the shells of live pteropods dissolve rapidly once surface waters become under-saturated with aragonite. When fourteen live sub-arctic pteropod Clio pyramidata were subjected to a level of under-saturation similar to what researchers predict for Southern Ocean surface waters in the year 2100, a marked dissolution occurred at the growing edge of the shell aperture within 48 hours (6). Etch pits formed on the shell surface at the apertural margin as the exterior prismatic layer peeled back, exposing the underlying aragonitic rods to dissolution. If these organisms cannot grow their protective shells, they may not survive in waters that become under-saturated with aragonite. This can cause a gap in the food chain, forcing the species of fish that depend on pteropods to search elsewhere for food and if unsuccessful die.
Fluctuations in plankton reduce survival of young cods
The Atlantic cod (Gadus morhua L.) has been overexploited in the North Sea since the late 1960s and great concern has been expressed about the decline in cod biomass and recruitment (11). A recent study shows that in addition to the effects of overfishing, fluctuations in plankton have resulted in long-term changes in cod recruitment (11). Taking a closer look at the causes of the fluctuations in the plankton ecosystem, scientists have found climate change at the root. Increased water temperature is having negative effects on the abundance and size of plankton.
Examining the long-term monthly changes in the plankton ecosystem of the North Sea and Skagerrak between the years 1958-1999 revealed clear distinctions between 1963-1983 and both of the surrounding periods of 1958-1962 and 1984-1999. The years 1963 to 1983 were characterized by a high abundance of prey for larval cod as well as a high mean size of calanoid copepods (12). In twelve of the 21 years from 1963 to 1983, cod recruitment (one-year-olds) in the North Sea was high, paralleling the positive anomalies in the plankton ecosystem. Cod recruitment, however, decreased from the mid-1980s, coincident with unfavorable changes in the plankton ecosystem, compared to 1963-1983 (12). Consistent with the conclusions of another recent study relating the survival of larval haddock to the timing of the phytoplankton bloom (12), these findings explain the increase in recruitment and therefore cod biomass during 1963-1983, as a consequence of a plankton population highly favorable for the survival of larval/juvenile cod.
A long-standing hypothesis contends that the abundance of fish year-classes is determined by food availability during the critical period of larval development (13). This has been supported for the survival of larval cod which were shown to depend on three key biological parameters of their prey; the mean size of the prey, seasonal timing and abundance (12). Examination of variables contributing to the first principal component of plankton showed that the relationship between plankton and cod is dependent on changes in the mean size of calanoid copepods. However, the mean size of calanoid copepods decreased by a factor of two after the beginning of the 1980s (12). The diminution in the mean size of calanoid copepods may be a consequence of major changes in the community structure of calanoid copepods recently detected in the northeast Atlantic and attributed to increasing temperature.
The Bigger Picture
Biodiversity Loss
Climate change has been found to affect all ocean organisms, impact the composition of marine communities and alter ecosystem function (14). Declining fish populations, as a result of climate change, contributes to the marine biodiversity loss we are experiencing today. These changes in marine biodiversity are directly caused by exploitation, pollution, and habitat destruction or indirectly through climate change and related perturbations of ocean biogeochemistry (15). Regional ecosystems such as estuaries (16), coral reefs (17) and coastal and oceanic fish communities (18) are rapidly losing populations, species or entire functional groups. By combining data from sources ranging from small-scale experiments to global fisheries, it can be concluded that there is a positive relationship between diversity and ecosystem functions and services.
Scientists have compiled long-term trends in regional biodiversity and services from a detailed database of 12 coastal and estuarine ecosystems and other sources. The trends of 30 to 80 economically and ecologically important species per ecosystem have been examined. Records over the past millennium revealed a rapid decline of native species diversity since the onset of industrialization (15). Like the experiments predicted, systems with higher regional species richness appeared to be more stable, showing lower rates of collapse and extinction of commercially important fish and invertebrate taxa over time (15). In large marine ecosystems, the rate of fisheries collapse has been accelerating over time, with 29% of currently fished species considered collapsed in 2003 (19). Despite large increases in global fishing effort, cumulative yields across all species and large marine ecosystems declined by 13% or 10.6 million metric tons since passing a maximum in 1994 (15). These collapses of large marine ecosystem fisheries occurred at a higher rate in species-poor ecosystems compared with species-rich ones. Also, the rates of recovery, defined as any post-collapse increase above the 10% threshold, were positively correlated with fish diversity (15).
Why does all this matter? As previously mentioned, the concern goes beyond conservation or preservation. Marine ecosystems provide a wide variety of goods and services, including vital food resources for billions of people. Alarmingly, there is an ongoing erosion of marine diversity and it appears to be accelerating on a global scale (15). The elimination of locally adapted populations and species not only impairs the ability of marine ecosystems to feed a growing human population but also sabotages their stability and recovery potential in a rapidly changing marine environment.
Unique Vulnerability of Indigenous Peoples to Climate Change
The Amazon
The global indigenous population of approximately 300 million people is composed of about 5,000 distinct indigenous cultures worldwide, living in every climate from the Arctic Circle to the tropical rain forest. Although Indigenous Peoples make up only 4 percent of the world’s population, they represent 95 percent of the world’s cultural diversity (20). An estimated 10 million Indians were living in Amazonia about five hundred years ago. Today there are fewer than 1 million indigenous peoples left in Amazonia.
The Amazon is home to nearly half of the world’s species of plants and animals, and also to about 1 million indigenous peoples of 400 different indigenous groups, all of whom are highly vulnerable and struggling to adapt to the changes caused by climate change (21). Deforestation and shifting temperature patterns are making the Amazon region drier and hotter. Since the mid-1970s, all tropical rainforest regions have experienced a strong warming at the mean rate of 0.26 + 0.05°C per decade, in synchrony with a global rise in temperature that has been attributed to the anthropogenic greenhouse effect (22). This change in temperature has serious implications for small indigenous cultures.
Research shows climate change may lead to large-scale redistribution of global catch potential, with an average drop of up to 40% in the tropics (4). Could the fish decline in this region can be attributed to similar responses seen in other fish populations around the world? If so, the consequences would be severe. For small, indigenous cultures, there are few alternatives should a critical resource become scarce. These are people living a way of life that has gone nearly unchanged for centuries. When a change in their world occurs, it’s never small.
Consider the Kamayurá tribe. For centuries it has depended on fish protein from jungle lakes and rivers. But things are changing rapidly. Chief Kotok, who like all of the Kamayurá people goes by only one name, said that men can now fish all night without a bite in streams where fish used to be abundant; they safely swim in lakes previously teeming with piranhas (23). To make do without fish, Kamayurá children are eating ants on their traditional spongy flatbread, made from tropical cassava flour. “There aren’t as many around because the kids have eaten them,” Chief Kotok said of the ants (23). Sometimes members of the tribe kill monkeys for their meat, but, the chief said, “You have to eat 30 monkeys to fill your stomach (23).”
But, of course, the fish are merely the canaries in the mine. The Kamayurá are feeling the heat of an interconnected ecosystem. Change one thing, like the temperature, and all manner of things change. So along with the declining in fish populations, the Kamayurá tribe’s agriculture has suffered as well. For centuries, the Kamayurá planted their summer crops when a certain star appeared on the horizon. Chief Kotok recalled, “When it appeared, everyone celebrated because it was a sign to start planting cassava since the rain and wind would come (23).” However, starting seven or eight seasons ago, the star’s appearance was no longer followed by rain, an ominous divergence, forcing the tribe to adjust its schedule (23). This has cause an ever-shifting game of trial and error. In 2008, the families had to plant their cassava four times because it died in September, October and November due to lack of moisture in the ground (23). The planting finally took, but not until December.
According to the Intergovernmental Panel on Climate Change, up to 30 percent of animals and plants face an increased risk of extinction if global temperatures rise just two degrees Celsius (23). However, as the Kamayurá tribe has shown, global climate change also brings about the risk for cultural extinction. Languages, art and traditions may also die with the fish.
We’re All in Hot Water Together
Let’s not argue whose fault this is, the planet’s cycle or man’s industrialization. The truth is the planet is changing. So can we do anything besides watch it change? We can. Reducing greenhouse gases is a smart approach, regardless of the cause of the warming. If it’s a natural cycle, our own emissions are only likely to speed it up, making our adaptation to the changes harder. And if we’re the cause of the trouble, altering our ways might slow the spread of change even more quickly, giving us, and the coral, time to adjust.
We can make better shopping decisions, too, particularly when it comes to fish. Avoid species classified as overfished and look for new sustainable farming labels at your grocer. Some people know that fish farming can add to water pollution. But it can also take from the food supply. Currently, salmon farmers must provide four pounds of feed fish for every pound of salmon they produce. But new technologies have been developed that bring the salmon to feed ratio down to a more sustainable 1:1 level (24).
The world loves fish. And if it wants to continue doing so, we’re going to have to change faster than the climate does.
References
1. “Fish Consumption and Aquatic Ecosystems | World Resources Institute.” World Resources Institute | Global Warming, Climate Change, Ecosystems, Sustainable Markets, Good Governance & the Environment. Web. 18 Apr. 2010. <http://www.wri.org/publication/content/8224>.
2. Portner, Hans O., and Rainer Knust. “Climate Change Affects Marine Fishes Through the Oxygen Limitation of Thermal Tolerance.” Science 315 (2007): 95-97. Print.
3. Cheung, William W., Vicky W. Lam, Jorge I. Sarmiento, Kelly Kearney, Reg Watson, and Daniel Pauly. “Projecting Global Marine Biodiversity Impacts Under Climate Change Scenarios.” Fish and Fisheries 10 (2009): 235-51. Print.
4. Cheung, William W., and Vicky W. Lam. “Large-scale Redistribution of Maximum Fisheries Catch Potential in the Global Ocean under Climate Change.” Global Change Biology 16 (2009): 24-35. Print.
5. Perry, Allison., Paula J. Low, Jim R. Ellis, John D. Reynolds. “Climate Change and Distribution Shifts in Marine Fishes.” Science 308 (2005): 1912-1915. Print.
6. Orr, James C. Et at. “Anthropogenic Ocean Acidification Over the Twenty-first Centry and Its Impact on Calcifiying Organsims.” Nature 437 (2005): 681-86. Print.
7. Hoegh-Guldberg, O., P. J. Mumby, A. J. Hooten, R. S. Steneck, P. Greenfield, and E. Gomez. “Coral Reefs Under Rapid Climate Change and Ocean Acidification.” Science 318 (2007): 1737-742. Print.
8. J.A. Kleypas, C. Langdon, Coast. Estuar. Stud. 61,73 (2006)—11.Print.
9. F. Moberg, C. Folke, Ecol. Econ. 29, 215 (1999) 11. Print.
10. Kobayashi, H. A. Growth Cycle and Related Vertical Distribution of the thecosomathous pterpod Spiratella “Limacina” helicina in the Central Arctic Ocean. Mar. Biol. 26, 295-301 (1974) 10. Print.
11. Cook, R. M., Sinclair, A. & Stefansson, G. Potential collapse of North Sea cod stocks. Nature 385, 521-522 (1997) 12. Print.
12. Beaugrand, Gregory, Keith Brander, J. Alistair Lindley, Sami Souissi, and Philip C. Reid. “Plankton Effect on Cod Recruitment in the North Sea.” Nature 426 (2003): 661-64. Print.
13. Munk, P. Pray size spectra and prey availability of larval and small juvenile cod. J. Fish Biol. 51 (suppl. A), 340-351 (1997). Print.
14. Brown, C. et al. “Effects of Climate-driven Primary Production Change on Marine Food Webs: Implications for Fisheries and Conservation. Global Change Biology (2009): 1-17. Print.
15. Worm, Boris, Edward B. Barbier, and Nicola Beaumont. “Impacts of Biodiveristy Loss on Ocean Ecosystem Services.” Science 314 (2006): 787-90. Print.
16. H. K. Lotze et al., Science 312, 1806 (2006). Print.
17. J. M. Pandolfi et al., Science 301, 955 (2003). Print.
18. B. Worm, M. Sandow, A. Oschlies, H. K. Lotze, R. A. Myers, Science 309, 1365 (2005). Print.
19. R. Froses, K. Kesner-Reyes, Impact of Fishing on the Abundance of Marine Speices [ICES Council Meeting Report CM 12/L:12, International Council for the exploration of the Sea (ICES), Copenhagen, Denmanrk, 2002]. Print.
20. Sobrevila, Claudia. “The Role of Indigenous Peoples in Biodiversity Concervation: The Nature of Often Forgotten Partners.” The World Bank (2008): 10-34. Print.
21. Gonzalo, Oviendo. “Indigenous Peoples and Climate Change.” European Parliament (2009): 11-13. Print.
22. Malhi, Yadvinder, and James Wright. “Spatial Patterns and Recent Trends in the Climate of Tropical Rainforest Regions.” The Royal Society 359 (2004): 311-29. Print.
23. Rosenthal, Elisabeth. “An Amazon Culture Withers as Food Dries Up.” The New York Times. Web. 5 Mar. 2010. <http://www.nytimes.com/2009/07/25/science/earth/25tribe.html?_r=1>.
24. “Salmon Farms, Sustainable Salmon Farming - Chile.” Cwirthy
Frozen Atlantic Salmon. Web. 20 April 2010. <http://www.cwirthy.com/sustainable-salmon-farming-in-chile.php>.