Determining the Missing Link: PGRN and TDP-43 in FTDP-17
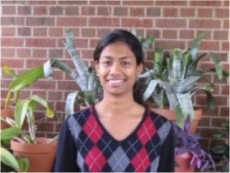
Madhavi Senagolage and Peter Sullivan
Download PDF
Abstract
Frontotemporal dementia with Parkinsonism linked to chromosome 17 (FTDP-17) is a neurodegenerative disease resulting in altered personality, memory impairment, and executive dysfunction. Currently, two genes, MAPT and PGRN, have been linked to FTDP-17. Several mutations in PGRN lead to a null allele and subsequently produce FTDP-17 symptoms without tau-positive inclusions. C-terminally cleaved TDP-43 was determined to be the major component of the inclusions in PGRN induced FTDP-17. Although PGRN and TDP-43 both have a role in pathogenesis, the relationship between the two is not clear. We propose that a knockdown of PGRN expression will increase the amount of caspase dependent cleavage of TDP-43 through a signal transduction pathway. We propose two possible candidate signal transduction pathways that PGRN may be using to regulate TDP-43. We will evaluate the involvement of TNF-R1 and MAPK/ERK signal transduction pathways in caspase dependent cleavage of TDP-43. We will also evaluate the relationship between PGRN and TDP-43 through PGRN knockout mice. This study will contribute to understanding the molecular mechanisms of FTDP-17, possible early diagnosis, and possible preventive methods for FTDP-17.
Background
Frontotemporal Dementia with Parkinsonism linked to Chromosome 17 (FTDP-17) is a devastating and complex neurodegenerative disease named in 1996 during a conference called to differentiate this dementia from others (Foster et al., 1997). Symptoms of FTDP-17 include executive dysfunction, altered personality and behavior, aphasia, Parkinsonism, and memory impairment (Boeve and Hutton 2008, Snowden et al., 2001). FTDP-17 varies from other dementias by its high prevalence in younger populations. It is the second most common form of dementia in people under 60 years old (Brun et al., 1994), which is about 10 years younger than the average sporadic onset of dementia from Alzheimer’s disease (AD) (Götz and Ittner 2008). FTDP-17 can be either sporadic or familial, with about 35-50% being familial (Chow et al., 1999). Through studies of families with high prevalence of FTDP-17, a mutation on chromosome 17 was found to be linked to the onset of dementia (Foster et al., 1997). This mutation was tracked to the MAPT gene, encoding the protein tau (Hutton et al., 1996). This discovery led to further research of the tau protein, also associated with AD, and the mutations that altered the function of tau.
The tau protein is found primarily in neurons of the central nervous system and help to stabilize microtubules through two mechanisms: isoforms and phosphorylation (Zhou, Yu, and Zou 2008). Tau has six isoforms with either three binding domains (3R) or four binding domains (4R) (Hong et al., 1996). 4R isoforms have a greater affinity for binding microtubules, though the ratio of 4R to 3R isoforms is about 1 (Goedert & Jakes, 1990). 3R and 4R isoforms are determined by the alternative splicing of exons 2, 3, and 10 in the MAPT gene (Zhou, Yu, & Zou 2008). Hyperphosphorylated tau has been found in intracellular inclusions in the neurons of the central nervous system (Lee, Goedert, & Trojanowski, 2001). These inclusions are a result of mutations in the MAPT gene causing self-assembly of the tau protein or a high 4R to 3R isoform ratio (Hong et al., 1998). Over forty FTDP-17 causing mutations have been discovered in MAPT (Goedert et al., 2005, Hutton et al., 1998). While the role of tau in FTDP-17 helps to understand the pathology, tau-positive FTDP-17 only accounts for 40% of familial FTDP-17 and does not fully explain the cause of FTDP-17 (Baker et al., 2006).
Another cause of FTDP-17 was later determined to be caused by mutations of the PGRN gene encoding progranulin, a growth factor known to be involved in several other neurodegenerative diseases (Baker et al., 2006). Mutations in PGRN generate tau-negative inclusions in the frontotemporal cortex, temporal neo cortex, and hippocampus (Shankaran et al., 2008), but these inclusions do not contain the protein progranulin either, suggesting that progranulin is not being synthesized (Baker et al., 2006). Discovery of over thirty-five mutations in PGRN have determined that PGRN mutations result in a null allele and the resulting mRNA is degraded by nonsense-mediated decay (Baker et al., 2006, Maquat, L. 2004). Investigation into the components of PGRN related inclusions revealed the Transactive response DNA-binding Protein (TDP-43) as a major component (Neumann et al., 2006).
TDP-43 is encoded in the gene TARDBP and is involved in several neurodegenerative diseases including Amyotrophic Lateral Sclerosis (ALS) and FTD (Li et al., 2004). Over-expession of TDP-43 alone has been shown to cause toxicity (Zhang et al., 2009). TDP-43 is cleaved by a caspase resulting in ~25 kDa c-terminal fragments, which aggregate to form inclusions (Neumann et al., 2006, Igaz et al., 2009). This relationship suggests that increased caspase will increase the cleavage of TDP-43, causing more aggregates (Zhang et al., 2007). In addition, evidence suggests PGRN regulates caspase, and therefore indirect regulates TDP-43 (Zhang et al., 2007). However, other studies have reported contradictory findings, suggesting that PGRN levels do not regulate caspase activity and therefore does not regulate TDP-43 cleavage and accumulation (Dormann et al., 2009). Therefore, more evidence is required to understand the correlation between PGRN and TDP-43.
PGRN is an extracellular anti-apoptotic factor that protects neurons from premature death (Bateman & Bennet, 2009). Growth factors like PGRN use cell-signaling pathways to control cellular mechanisms. Therefore, We hypothesize that PGRN regulates TDP-43 cleavage in FTDP-17 through a signal transduction pathway. Tumor Necrosis Factor- receptor 1(TNF-R1) and MAPK/ERK are two possible pathways for PGRN to utilize in cell signaling (He et al., 2002, Zhang et al., 2002, Bateman & Bennet, 2009). Tumor necrosis factor (TNF) is a major moderator of apoptosis, inflammation and immunity. The interaction of TNF with TNF- receptor-1(TNF-R1) is found to activate several signal transduction pathways (Chen & Goeddel, 2002). This interaction triggers the release of an inhibitory protein silencer from TNF-R1’s intracellular domain (ICD), leading to the recruitment of many proteins including fas associated death domain (FADD). FADD activates caspases to cleave proteins, causing apoptotic cell death (Chen & Goeddel, 2002), thus making this signaling pathway a possible candidate for further investigation under PGRN deficient conditions. MAPK/ERK pathway recruits the G-protein Ras, Raf, MEK and the ERK kinasses (Kolch, 2000). Activation of these kinases turns on many transcription factors including c- myc (Zhang et al., 2002). C-myc controls Bcl-12 family proteins (Eichen et al., 2001). Bcl2 family consists of both anti -apoptotic and pro-apoptotic proteins. Bax, which is a pro-apoptotic Bcl2 family member promote cell death by protein cleavage and gets activated when anti-apoptotic Bcl2 levels, are too low. Therefore, it is possible that in the absence of PGRN, c-myc indirectly increase Bax activation thus protein cleavage by caspases, making MAPK pathway an interesting area of focus in the absence of PGRN.
We predict that in the absence of PGRN, one or both of these signaling pathways becomes activated to regulate the TDP-43 cleavage, eventually leading to cell death by apoptosis. Studies will be done both in vitro and in vivo. Our study will advance the understanding of the underlying molecular mechanisms of the relationship between PGRN levels and TDP-43 as well as present a possible therapeutic approach.
Significance
Broader Relevance
Dementias are a major health and social concern in our aging society. According to the World Alzheimer report for 2010, the global cost of Alzheimer’s and related dementias is estimated to be $604 billion USD (World Alzheimer report, 2010). Frontotemporal dementia and Parkinsonism linked to chromosome 17 is considered to be the second most common neurodegenerative disease among people less than 60 years old (Baker et al., 2006). Advances in the field over the past decade have expanded our knowledge about FTDP-17. Nonetheless, the prevalence and incidence still remain unknown (Wszolek et al., 2006). It is possible that requirement of characteristic clinical and pathological features and molecular genetics analysis make identification of the disease more challenging. Therefore, it is necessary to examine molecular mechanisms and pathological features underlying FTDP-17, which might help to develop therapeutic approaches to FTDP-17.
Our study will also help train undergraduate and graduate researchers by exposing them to experiments ranging from smaller molecular projects to complex experiments. This study will attract a diverse range of young researchers and train them to be competent scientists in the field.
Intellectual Merit
Our study focuses on understanding both general pathology and molecular mechanisms underlying FTDP-17. This study will also support or refute contradictory evidence (Zhang et al., 2007, Dormann et al., 2009) that is presented in the field regarding the relationship between PGRN and TDP-43. Furthermore, insight into the exact molecular mechanism will illustrate the relationship between PGRN and TDP-43. This study will also provide further evidence to distinguish the connection between PGRN and TDP-43 in vertebrate and invertebrate models and link them to human pathology. An intellectual perceptive of these pathways may lead to the development of clinical diagnosis methods in the future.
Specific Aims
The ultimate goal of our study is to advance the clinical diagnosis of FTDP-17 by understanding molecular mechanisms and pathology. We propose the hypothesis that PGRN levels regulate TDP-43 cleavage through signal transduction pathways in FTDP-17.
Our aims for our hypothesis are as follows.
1. Evaluation of PGRN dependent TDP-43 cleavage by caspases using TNF-R1 signal transduction pathway:
Using H4 neuroglioma cell line, we will measure the caspase 8 activation followed by caspase 3 activation in the absence of PGRN. We will also determine the change in levels of TNF, which will indicate the activation of TNF pathway leading to apoptosis (Chen, G. et al., 2002). We will evaluate if other signal transduction molecules such as FADD and Fas involved in TNF-R1 pathway, get activated due to the inflammation response caused by absence of PGRN. Subsequently, we will also assess how inhibition of those specific signal transduction molecules will affect TDP-43 cleavage by caspase 3.
2. Evaluation of PGRN dependent TDP-43 cleavage by caspases using MAPK/ERK signaling pathway:
The caspase 9 and caspase 3 activation in the PGRN deficient H4 nueroglioma cells will be analyzed. Then, we will be assessing the reduced phosphorylation of MAPK/ERK pathway due to lack of PGRN, which we predict will promote apoptosis by activating Bax and cause TDP-43 cleavage, thus apoptotic cell death. The involvement of signal transduction molecules and how their absence might reverse or affect TDP-43 cleavages by caspase 3 will also be assessed.
3. To demonstrate that PGRN knockout mice show caspase dependent accumulation of TDP-43 fragments:
We will assess the accumulation of caspase cleaved TDP-43 fragments by means of immuno-electron microscopy and western blotting using sections taken from the frontal cortex and hippocampus of the model organism for both progranulin and TDP-43 to determine the interaction between the proteins in a mouse model. By using a mouse model, we will determine if factors, not seen in the cell model, affect the levels of PGRN and TDP-43. As the mouse model is more complex than a cell model, the mouse model will more accurately represent FTDP-17 in humans.
Research Design and Methods
1. Evaluation of PGRN dependent TDP-43 cleavage by caspases using TNF-R1 signal transduction pathway.
Rationale: Progranulin is capable of inhibiting the inflammation in cells by TNF-alpha mediated TNF activation (Zhu et al., 2002). Previous studies have shown an increase in TNF levels in PGRN deficient mice (Ying et al., 2009). Therefore, we believe that the absence of PGRN activates the TNF signaling pathway, thus caspase activation, which leads to cleavage of TDP-43 and apoptosis respectively.
Design and Method
The activation of TNF-R1 pathway in PGRN deficient H4 neuroglioma cells:
PGRN deficient H4 neuroglioma cells: H4 neuroglioma cells will be grown in Opti-Mem plus 10% FBS and 1% penicillin-streptomycin and will be passaged every 3-5 days based on confluence of a 95% (Zhang et al., 2007). The lysates will be prepared 48 hours after PGRN small interfering RNA (siRNA) transfection for western blot analysis unless mentioned specifically. Rabbit polyclonal anitibody for PGRN (Zhang, et al., 2007) will be used to detect the presence or absence of progranulin.
The activation of TNF pathway:
The activation of TNF pathway will be detected by several methods. First, we will identify whether cells express higher levels of TNF as an indication of activation of TNF pathway that leads to apoptosis by cleavage of TDP-43. TNF levels will be detected from 0-48 hrs within intervals of 6hrs after siRNA transfection, using western blot analysis. Secondly, as cleavage of caspases will provide evidence for their activation we will identify the activation of caspases by comparing western blot analysis of cleaved caspases in H4 cells and that of control H4 cells. We will also detect cleaved TDP-43 in the absence of PGRN. Antibodies, rabbit polyclonal anti-caspase-3 and rabbit polyclonal anti-caspase-8 (Dormann et al., 2009) for full length and cleaved caspase 3 and 8 will be used respectively. Rabbit polyclonal antibody for TDP-43 (Zhang, et al., 2007) and TDP-43 fragments will be used to detect the cleavage of TDP-43. β- actin will serve as protein loading control and be recognized by mouse monoclonal anti- β-actin antibody.
TDP-43 localization changes:
Using immunocytochemistry (ICC) we will also assess any changes in localization of TDP-43 under PGRN knockdown conditions in H4 cells. The cells will be grown on cover slips and then will be then exposed to primary antibody, rabbit polyclonal antibody to TDP-43 and secondary antibody respectively (Dormann et al., 2009). After staining with molecular probe, TOPRO-3 iodide (Dormann et al., 2009), the nuclear localization changes or any changes in localization, will be detected by confocal microscopy.
Nuclear changes and apoptotic body formation:
Next, we will also quantify apoptotic cells after PGRN knock out using ethidium bromide and acridine orange (EB/AO) with 96-well plates staining method (Ribbel et al., 2005) to visualize characteristics of apoptosis such as nuclear changes and apoptotic body formation (Spector et al., 2006) We will count cells under a fluorescence microscope and quantify cells that have undergone apoptosis.
FADD dependent activation of caspases and apoptosis: Finally, we will demonstrate the activation of TNF-R1 signal transduction pathway by inhibiting FADD associated apoptosis (Rosenthal et al., 2003) by expressing dominant negative FADD (FADD-DN) and assess the effects on caspase activity and TDP-43 cleavage. Also, changes in localization of TDP-43 using immunocytochemistry and cells that have undergone apoptosis will be assessed by methods described earlier.
Prediction: We anticipate that TNF pathway gets activated as a result of PGRN knockdown. This will be demonstrated by activation of TNF, caspases 3 and 8 and also cleavage of TDP-43. We also expect to see localization changes in fragmented TDP-43 in H4 cells. By blocking signal transduction molecules in the TNF-R1 pathway we expect to see opposite results from the above. The inhibition of FADD should prevent recruitment of caspase 8 thus proteolysis of caspase 3 and cleavage of TDP-43 that leads to apoptosis. If evidence will not support our hypothesis that, TDP-43 cleavage occur through activation of TNF-R1 signal transduction pathway under PGRN deficient conditions, then it can be concluded that another pathway like MAPK/ERK signal transduction pathway is used to activate caspases thus cleavage of TDP-43 and apoptosis.
2. Evaluation of PGRN dependent TDP-43 cleavage by caspases using MAPK/ERK signaling pathway:
Rationale: MAPK/ERK pathway is a chain of proteins in the cell that communicates a signal from a receptor on the surface of the cell to the DNA in the nucleus of the cell (Kolch, W., 2000, Zhang et al., 2002). When the growth factors like, PGRN is present it binds to the receptor in cell membrane thus activation the MAPK/ERK pathway that will promote cell proliferation by phosphorylation of transcription factor c-myc (Schönwasser et al., 1998). C-myc is also known to phosphorylate Bcl-2 family proteins like Bcl-2 and Bcl-XL thus suppress apoptosis (Nunez, G. et al., 1990, Eischen C. et al., 2001). Deposhphorylation of myc and deactivation of Bcl-2 can lead to activation of pro-apoptotic agents like Bax and Bad (Nunez, G. et al., 1990). Apoptosis can be induced by absence of growth factors (Nunez, G. et al., 1990). We believe that the absence of PGRN prevents the phosphorylation/activation of c-myc thus down regulate Bcl-2 and up-regulate Bax activity leading to TDP-43 cleavage and apoptosis.
Design and Method
The activation of MAPK/ERK pathway in PGRN deficient H4 neuroglioma cells:
PGRN deficient H4 neuroglioma cells: Cells will be created as described earlier in aim 1.
Involvement of MAPK/ERK pathway and Bcl-2 family proteins:
We will be measuring the involvement of MAPK/ERK pathway in several ways. First, we will identify the activation of caspases and cleavage of TDP-43 by comparing western blot analysis of caspase activity in PGRN knockout H4 cells and control H4 cells as described above. Other than caspase3 activation, Rabbit anti-caspase-9 antibody (Hu Y. et al., 1998) will be used to detect caspase9 activation (Knudson et al., 1997, Hu Y. et al., 1998, Janssen et al., 2007).
Apart from that we will also be using antibodies to detect signal transduction molecules such as c-myc and MAPK using western blot analysis. In the absence of PGRN, phosphorylated c-myc should not be detected. We will also measure the Bcl-2 levels and Bax protein levels to determine Bax dependent activation of caspases and cleavage.
We will also evaluate how alteration of MAPK pathway molecules will affect cleavage of TDP-43 if PGRN is using this pathway for molecular signaling by inhibiting MAPK pathway signaling molecules in several ways. First, Bax will be inhibited by over-expressing Bcl-XL (Yin et al., 1993, He, L. et al., 2003) and we expect to see no TDP-43 cleavage by caspase 3, no caspase 9 activation and no change in TDP-43 localization and lower apoptotic cell death. Secondly, RNAi (small interefereing RNA) will be used to inhibit MAPK, ERK and Ras, in the presence of PGRN, and we expect to see higher Bax levels, cleaved TDP-43, cleaved caspases and apoptotic cell death. This will further confirm the usage of MAPK pathway by PGRN.
TDP-43 localization and apoptotic cell death:
Thirdly, using confocal microscopy we will measure localization changes of TDP-43 using the molecular probe, TOPRO-3 iodide, as described above. Quantification of apoptotic cells will also be done as described in aim 1a.
Prediction: We predict that the absence of PGRN prevents up-regulation of anti apoptotic Bcl-2 by MAPK pathway and cause cleavage of TDP-43 protein and eventually apoptotic cell death. If evidence will not support our hypothesis that MAPK/ERK molecules do not get phosphorylated thus prevent bcl-2 anti-apoptotic actions and activate Bax promoting apoptosis, it can be predicted that another pathway is being used or compromised.
These studies will further help our understanding about the relationship between PGRN and TDP-43 or support/refute already available explanations in the field.
3. To demonstrate that PGRN knockout mice show caspase dependent accumulation of TDP-43 by means of western blot analysis of PGRN, and TDP-43.
Rationale: Previous research has shown contradictory data regarding PGRN levels’ affect on the caspase cleavage of TDP-43 into 25 kDa fragments (Zhang Y. et al., 2007, Dormann et al., 2009). We believe that TDP-43 aggregation will be regulated indirectly by PGRN through caspase cleavage. To test this hypothesis in a way comparable to the human condition of FTDP-17, we will produce mice PGRN knockout models. If TDP-43 cleavage is regulated by PGRN levels, then an increase in aggregation of TDP-43 fragments will be seen in the frontal cortex and hippocampus neurons, producing toxicity and FTDP-17 like symptoms. These symptoms can then be compared to previous literature of FTDP-17 mice models (Tatebayashi, Y. et al., 2002) Western blot analysis, immuneflorescence and immuno-electron microscopy will be used to quantify and visualize PGRN and TDP-43. Understanding of the relationship between PGRN and TDP-43 may help to establish methods to determine FTDP-17 pathogenesis during earlier stages of disease progression.
Design and Method
Knockout mice will be generated by replacing the PGRN gene with a null allele. Since many of the common mutations seen in FTDP-17 patients create null alleles, a mutation of PGRN will be used. Q125X is a nonsense mutation found in familial FTDP-17 patients and produces a null allele of PGRN, compromising the full production of progranulin protein (Baker et al., 2006). The cDNA construct that will be used to produce the transgenic mice will use myc to bind the vector to DNA in the XhoI site, FLAG as a protein tag in the NotI site, alpha-calcium-calmodulin-dependent kinase II (CaMKII) as the promoter (Tatebayashi et al., 2002), and Q125X PGRN. This vector will be used to transfect mouse embryonic stem cells. The resulting mice will then be cross bred to produce homozygous Q125X PGRN mice and heterozygous Q125X PGRN mice. To ensure that the expressed levels of progranulin are in fact lowered in the transgenic mice, northern blot analysis will be used as described by Baker et al., 2006. We expect to see less PGRN mRNA produced in the Q125X heterozygous and homozygous mice than the WT mice. This would support preexisting findings and allow our procedure to continue. Alternative findings would suggest that the transfection did not work properly.
After confirming the levels of progranulin expressed in each mouse model, the relative levels of TDP-43 can be evaluated through immuno-electron microscopy using rabbit anti-PGRN and rabbit polyclonal anti-TDP-43 for determining if TDP-43 aggregation has increased and anti-CD68 as a control to ensure that equal loading has taken place. Brain sections will be taken from the frontal cortex and hippocampus. These procedures follow as outlined by Baker et al., 2006. The brain sections can then be visualized by electron microscopy.
In addition to microscopy, western blot analysis will be used to more accurately quantify the levels of TDP-43. Procedures will follow those outlined by Tatebayashi et al., 2002. Western blots can then be analyzed by a computer-linked LAS-1000 Bio-Imaging Analyzer (Fuji) using the program IMAGE GAUGE 3.0 (Fuji) to quantify the amounts of TDP-43 and progranulin in the frontal cortex and hippocampus cells. TDP-43 will also be analyzed to be either cleaved (25 kDa) or non-cleaved (43 kDa).
Prediction: Mice with lower levels of PGRN mRNA will produce less progranulin and in turn have less caspase-dependent cleavage of TDP-43. This will be evident if TDP-43 immunohistochemistry and western blot show an increase in the amount of intracellular inclusion of TDP-43 in microscopy or an increase in the amount of insoluble 25 kDa TDP-43 fragments in western blotting. If we do not see an increase in the levels of TDP-43 mRNA, then progranulin may not be the protein causing the caspase to cleave TDP-43. If no 25 kDa TDP-43 is found by the western blot, then no cleavage occurred, though progranulin may still regulate levels of TDP-43.
These studies will further our understanding about the relationship between PGRN and TDP-43 in a vertebrate model and may provide possible methods for early diagnosis of FTDP-17. This study will also support/ refute contradictory findings from the field.
References
1. Alzheimer’s disease international, World Alzheimer report, (2010), Chicago, IL, USA and London, UK, Retrieved from :http://www.alz.co.uk/research/files/WorldAlzheimerReport2010ExecutiveSummary.pdf
2. Baker M, Mackenzie IR, Pickering-Brown SM, Gass J, Rademakers R, Lindholm C, Snowden J, Adamson J, Sadovnick AD, Rollinson S, Cannon A, Dwosh E, Neary D, Melquist S, Richardson A, Dickson D, Berger Z, Eriksen J, Robinson T, Zehr C, et al. (2006) Mutations in progranulin cause tau-negative frontotemporal dementia linked to chromosome 17, Nature 442:916 –919. doi:10.1038/nature05016.
3. Bateman A. & Bennet H. (2009).The granulin gene family: from cancer to dementia, BioEssays 31:1245–1254. DOI 10.1002/bies.20090008.
4 .Boeve B. & Hutton,M. (2008) Refining Frontotemporal Dementia With Parkinsonism Linked to Chromosome 17, Arch. Neurol, 65 (4), 460-464.
5. Brun, A. et al. (1994). Clinical and neuropathological criteria for frontotemporal dementia. The Lund and Manchester groups. J. Neurol. Neurosurg. Psychiatry 57, 416–-418.
6. Chen, G., Goeddel, D. (2002) TNF-R1 signaling: A beautiful pathway. Science. 296, 1634-1635.
7. Chow, T. W., Miller, B. L., Hayashi, V. N. & Geschwind, D. H. (1999). Inheritance offrontotemporal dementia. Arch. Neurol. 56, 817–-822.
8. Dormann D, Capell A, Carlson A., Shankaran S, Rodde R, Neumann M, Kremmer E, Matsuwaki T, Yamanouchi K, Nishihara Haass C.(2009), Proteolytic processing of TAR DNA binding protein-43 by caspases produces C-terminal fragments with disease defining properties independent of progranulin, Journal of Neurochemistry 110, 1082–1094. doi: 10.1111/j.1471-4159.2009.06211.x
9. Eischen C. et al. (2001). Bax Loss Impairs Myc-Induced Apoptosis and Circumvents the Selection of p53 Mutations during Myc-Mediated Lymphomagenesis. Milecular and Cellular Biology. 21(22), 7653-7662
10. Foster, N. et al. (1997), frontaltemporal dementia and Parkinsonism linked to Chromosome 17: A Consensus Conference. American Neurological Association 706-715.
11. Goedert, M., Jakes, R. & Crowther, R. A. (1999) FEBS Lett. 450, 306–311.
12. Goedert M. (2005) Tau gene mutations and their effects. Mov. Disord. 20, S45–S52.
13. Götz and Ittner (2008). Animal models o Alzheimer’s disease and frontotemporal dementia. Nature. 9, 532-544.
14. He, L., Perkins, G., Harris, J., Hung, M., Ellisman, M., Fox, D., (2003), Bcl-xL overexpression blocks bax-mediated mitochondrial contact site formation and apoptosis in rod photoreceptors of lead-exposed mice PNAS 100 (3) 1022-1027. doi:10.1073/pnas.0333594100.
15. Hong M, Zhukareva V, Vogelsberg-Ragaglia V, Wszolek Z, Reed L, Miller BI, Geschwind DH, Bird TD, McKeel D, Goate A, Morris JC, Wilhelmsen KC, Schellenberg GD, Trojanowski JQ, and Lee VM (1998) Mutation-specific functional impairments in distinct tau isoforms of hereditary FTDP-17. Science 282: 1914–1917.
16. Hu, Y., Benedict M., Wu, D., Inohara, N., Nunez, G., (1998), Bcl-XL interacts with Apaf-1 and inhibits Apaf-1-dependent caspase-9 activation, Proc. Natl. Acad. Sci. USA (95), 4386–4391
17. Hutton M, Baker M, Houlden H, et al. (1996). Genetic analysis of an Australian pedigree with autosomal dominant non-Alzheimer’s dementia. Am J Hum Genet;59(suppI):A221 (abstract) 37. Sanders J, Schenk W D , van Veen P. A fainily with Picks disease. Verh K Nederland Akad Wetensch 1939; section 2, part 38, no. 3
18. Hutton, M. et al., (1998). Association of missense and 5′‑splice‑site mutations in tau with the inherited dementia FTDP‑17. Nature 393, 702–705.
19. Igaz LM, et al. (2009) Expression of TDP-43 C-terminal Fragments in Vitro Recapitulates Pathological Features of TDP-43 Proteinopathies. J Biol Chem 284:8516–8524.
20. Janssen, K., Pohlmann, S., Jänicke U., Schulze-Osthoff, K., Fischer, U., (2007), Apaf-1 and caspase-9 deficiency prevents apoptosis in a Bax-controlled pathway and promotes clonogenic survival during paclitaxel treatment, doi:10.1182/blood-2007-02-073213
21. Knudson M. & Korsmeyer S. J., (1997), Bcl-2 and Bax function independently to regulate cell death, Nature Genetics 16, 358 – 363.
22. Kolch, W., (2000), Meaningful relationships: the regulation of the Ras/Raf/MEK/ERK pathway by protein interactions Biochem. J. 351, 289-305.
23. Lee VM, Balin BJ, Otvos L, Jr, Trojanowski JQ (1991) A68: A major subunit of paired helical filaments and derivatized forms of normal Tau. Science 251:675–678.
24. Lee, Goedert, & Trojanowski, (2001), Neurodegenarative tauopathies, Annl. Review of Neurosci. 24, 1121-1159.
25. Maquat, L. E. (2004) Nonsense-mediated mRNA decay: splicing, translation and mRNP dynamics. Nature Rev. Mol. Cell Biol. 5, 89–-99.
26. Neumann M, Sampathu D, Kwong L, et al.(2006). Ubiquitinated TDP-43 in frontotemporal lobar degeneration and amyotrophic lateral sclerosis. Science. 314(5796):130-133.
27. Nunez G.,London L., Hockenbery, D., Alexander, M., McKearn J.P., & Korsmeyer S.J. (1990), Deregulated Bcl2 gene expression selectively prolongs survival of growth factor-deprived hematopoietic cell lines. J Immunol ; 144: 3602–3610.
28. Ribbel D. Goldstein N, Norris D. & Shellman Y. (2005) A simple technique for quantifying apoptosis in 96-well plates, BMC Biotechnol. 5: 12 ; doi: 10.1186/1472-6750-5-12.
29. Rosenthal,S., Velena, A., Chou, F., Schlegel, R., Ray, R., Benton, B., Anderson, D., Smith, J., & Simbulan-Rosenthal, S.M. (2003), Expression of Dominant-negative Fas-associated Death Domain Blocks Human Keratinocyte Apoptosis and Vesication Induced by Sulfur Mustard, The Journal of Biological Chemistry 278 (10), 8531–8540.
30. Schönwasser et al. (1998) Activation of the Mitogen-Activated Protein Kinase/Extracellular Signal-Regulated Kinase Pathway by Conventional, Novel, and Atypical Protein Kinase C Isotypes. Molecular and Cellular Biology. 18(2), 790-798.
31. Shankaran S. et al (2008). Missense Mutations in the Progranulin Gene Linked to Frontotemporal Lobar Degeneration with Ubiquitin-immunoreactive Inclusions Reduce Progranulin Production and Secretion. The J. of Biological Chemistry 283 (3), 1744–1753
32. Snowden J.S. et al., (2001). Distinct behavioral profiles in frontotemporal dementia and semantic dementia. J. Neuro. Neurosurg. Psychiatry. 70, 323-332
33. Spector et al (2006), Acridine Orange/Ethidium Bromide (AO/EB) Staining to Detect Apoptosis, Cold Spring Harb. Protoc.; 2006; doi:10.1101/pdb.prot4493
34. Tatebayashi, Y. et al. (2002) Tau filament formation and associative memory deficit in aged mice expressing mutant (R496W) human tau. Proceedings of the National Academy of Sciences 99 (21), 13896-13901.
35. Wszolek, Z., Tsuboi Y., Ghetti B., Pickering- Brown, S., Baba Y., Cheshire W. P. (2006) Frontotemporal dementia and Parkinsonism linked to chromosome 17 (FTDP-17) Orphanet Journal of Rare Diseases, 1:30. doi:10.1186/1750-1172-1-30.
36. Yin, F., Banerjee, R., Thomas, B., Zhou, P., Qian, L., Jia, T., Ma, X., Ma, Y., Iadecola, C., Beal, M., Nathan, C. & Ding, A., (2009), Exaggerated inflammation, impaired host defense, and neuropathology in progranulin-deficient mice, The Journal of Experimental Medicine 207 (1) 117-128.
37. Yin, X., Oltvai Z., & Korsmeyer S., (1994), BH1 and BH2 domains of Bcl-2 are required for inhibition of apoptosis and heterodimerization with Bax, Nature 369, 321-323.
38. Zhang W. & Liu H. (2002), MAPK signal pathways in the regulation of cell proliferation in mammalian cells, Cell Research, 12 (1), 9-18.
39. Zhang Y., Xu, Y., Dickey, C., Buratti E., Baralle, F., Bailey R., Pickering-Brown, S., Dickson, D., & Petrucelli, L., (2007) Progranulin Mediates Caspase-Dependent Cleavage of TAR-DNA Binding Protein-43, The Journal of Neuroscience 27(39):10530 –10534.
40. Zhang et al. (2009). Aberrant cleavage of TDP-43 enhances aggregation and cellular toxicity. Proceedings of the National Academy of Sciences. 106(18), 7607-7612.
41. Zhou, Yu, and Zou. (2008) Alternative splicing of exon 10 in the tau gene as a target for treatment and tauopathies. BMC Neuroscience. 9 (suppl 2):s10 doi:10.1186/1471-2202-9-S2-S10
42. Zhu J, Nathan C, Jin W, Sim D, Ashcroft GS, Wahl SM, Lacomis L, Erdjument-Bromage H, Tempst P, Wright CD, Ding A (2002) Conversion of proepithelin to epithelins: roles of SLPI and elastase in host defense and wound repair. Cell 111:867– 878.