TRP’S Sensational Pathways: Unlocking Mysteries of Pain & Temperature
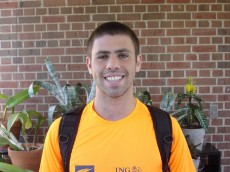
Jon Flaksman
Download PDF
Summary
The animal nervous system has evolved complex neural pathways to respond to a wide variety of stimuli, including chemical and physical pain, as well a range of harmless or noxious temperatures, but the mechanism behind these actions is poorly understood. The inability to perceive these sensations would make species survival impossible. Therefore, my lab explores the molecular mechanisms involved that determine whether an organism will feel pain or a change in the intensity of heat or cold. We discovered that different families of the transient receptor potential channel (TRP) mediate the responses to these stimuli by opening or closing their pores to extracellular calcium. Using household ingredients such as chili peppers, mustard oil and menthol, our lab further uncovered more detailed mechanistic models that serve to explain the reasoning to how and why animals can sense pain and changes in temperature. Furthermore, my lab determined the mechanisms of how TRP channels also serve as mediators to injury by causing inflammation. With this knowledge, TRP channels can serve as useful targets for novel analgesic drugs that treat a wide variety of tissue injury and degenerative conditions that promote inflammation and chronic pain.
At any given moment, we can feel a wide variety of environmental stimuli. The cold feeling that arises while eating an ice cream cone, the warmth of a hot sunny day, or even a painful sting from a bee. These sensations occur predominantly on the primary sensory neurons of the dorsal root ganglia (DRG) or trigeminal root ganglia (TG), which are capable of causing pain, heat, cold, and tissue damage1,2. In fact, electrophysiological studies have shown the existence of sensory neurons that can be excited by noxious heat, noxious cold, and pain via the activation of protein channels3. A subpopulation of primary afferent neurons shows that channels have specific thresholds. These thresholds determine whether a stimulus, such as heat, should elicit excitation and cause a sensation to occur or not3,4. Important information about nociception has been gained through analysis of natural products that mimic the sensations of hot and cold temperatures. Capsaicin is the main pungent ingredient found in chili peppers, which is associated with the burning, hot, and painful sensations that can be felt during their consumption. Other than its use in natural medicine and cultural cooking, capsaicin has grown to be widely used in the study of pain and heat5,27. Menthol, a plant product, is known to elicit a cool sensation. For this reason, it is used as a chief substance in the pursuit to understand the mechanistic processes of cold sensations5.
These products, along with other household ingredients, have been used in my lab in order to better understand the dynamics of pain and thermosensation. As the map of sensation is being unfolded, it is becoming apparent that specific stimuli activate their own respective protein channel in a variety of ways. It is currently understood that many key ion channels play their own roles in order to promote the specific sensations experienced by an organism. Some of the natural responses caused by excessive heat or cold are inflammation and tissue damage. In response to intense temperatures, there is an increase in proton abundance, which in turn sensitizes ion channels and makes tissues more prone to damage5. With the identification of these ion channels, our lab gained insight into the molecular knowledge of how signal transduction pathways work, in regard to different types of stimuli. Furthermore, the accumulated research about pain and thermosensation can be used in pharmacological studies, in order to develop novel analgesics that can target and treat chronic pain and inflammation caused by injury6.
Capsaicin Stepping Stone, Nociception
Capsaicin is the molecule present within hot chili peppers that elicits spicy and painful sensations when consumed. Capsaicin belongs to a group of compounds known as vanilloids. The hot sensation that accompanies taste during their consumption makes them a perfect model to research the mechanism of pain and heat. Bevan et al. conducted electrophysiological experiments in 1990 that confirmed that capsaicin excites sensory neurons, via the activation of ion permeable channels. For many years, the molecular mechanism of the elicited effect was unknown1. In 1993, James et al. proposed a model for pain perception that involved the activation of specific channels on sensory neurons. This model sparked my attention, and in 1997, my lab began performing a channel cloning technique. We used rat DRG, and based on calcium influx we attempted to find a protein channel that responded to treatment with capsaicin in vitro1. Using the results gained from this experiment, we discovered a DNA sequence encoding the receptor for a specific channel that mediates the calcium currents. We called this channel the vanilloid receptor 1 (VR1)1. We then compared this DNA sequence to other sequences found in the GenBank database. We then identified this channel as part of TRP channel family. With this new knowledge, it was then possible to begin uncovering the molecular mechanisms that underlie nociception.
Capsaicin Activates VR1 in a Pain Related Fashion
Using human embryonic kidney (HEK) cells and rat DRG, in vitro experiments were conducted using capsaicin and extracts from four other peppers. This was done to determine whether the elicited currents matched the potencies of the peppers themselves. We indeed saw a correlation in the currents observed. The more potent pepper extracts elicited stronger currents in VR1 channels1. This implicated that the VR1 channels respond linearly in their permeability to ions in response to the strength of a stimulus. Next, we performed a northern blot of different rat tissues, which indicated that VR1 channels are exclusively located in DRG and TG, and not in other internal structures such as the small intestine or the heart1. Additionally, immunocytochemical analyses were performed on rabbit
spinal cords and caudal brainstems that localized VR1 in afferent sensory neurons and in the caudate nucleus of TG2. These results further demonstrated that VR1 channels are specialized in the function of nociception.

Getting bit by a creature that secretes venom is not a pleasant experience. These creatures use venom as a defense mechanism to ward off their enemies. Modern pharmacology has been able to determine the types of molecules present in the venom of some creatures, but almost nothing is known about the mechanisms that play a role in producing pain7. Twenty-two venoms were assayed in order to detect activators of sensory neurons. Vanillotoxin 1 (VaTx1) is venom that excites sensory neurons, but in a less conventional way than what is normally thought of. Rather than increasing an ion channel’s permeability, VaTx1 inhibits Kv2.1 channels in a dose dependent manner7. Spiders are not the only organisms that excite sensory neurons through the inhibition of potassium channels. Hydroxy-α-sanshool (sanshool), the molecule present in Szechuan peppers, elicits a tingling feeling when consumed. These peppers have been used for their medicinal and culinary properties in traditional Asian and Native American cultures, yet the mechanism of their action is not well understood8. For example, in 1999, Bryant et al. suggested that sanshool activates sensory neurons that respond to cooling, heart or light touch. However, recently sanshool has been proposed to activate all sensory neurons8. Our lab performed whole-cell voltage-clamp recording methods, in order to determine the nature of the ionic current evoked by sanshool. It was observed that addition of sodium and calcium ion chelators did not cause a change in the current. However, when sodium ions were replaced with potassium ions, it was observed that the reversal potential shifted from a negative to a positive value.This suggests that sanshool excites sensory neurons through inhibition of potassium channels8.
Capsaicin and Heat
Because capsaicin causes a burning sensation along with the pain elicited when ingested, we believed that capsaicin and heat work in a similar molecular pathway. In order to test this, we conducted more in vitro experiments. In a dose dependent manner, when HEK cells were exposed to incremental molar values of capsaicin, along with a steady increase in heat, the currents evoked by the channels were nearly identical1,2. Once we understood that heat and pain work in a similar pathway, we wanted to delve deeper into understanding how temperature affects the VR1 channel. Xenopus oocytes transfected with VR1 were placed in an ambient bath (22.1°C) and no current was evoked, but when the bath temperature was raised to a high temperature (43°C), a strong current was evident1. This result gave evidence to the fact that specifically high temperatures activate the VR1 channel.
Connecting Pain and Heat In Vivo
Our lab now had evidence that VR1 functions in the pain and temperature pathway in Xenopus and mammalian cells. The next step was to confirm our previous in vitro results through in vivo experiments. VR1 deficient (VR1-/-) and wildtype (WT) mice were given a sugar solution to drink throughout four days. On the fifth day, the sugar solution was replaced with capsaicin. On the fifth day of the test, WT mice ceased drinking the water, while VR1-/- mice continued to drink the capsaicin solution, indicating that they did not feel any chemical pain5. Additionally, when the two groups of mice were subjected to a paw-licking test, they had capsaicin injected into their hind paws. WT mice commenced to lick their paw as a coping mechanism to deal with the pain, while VR1-/- mice showed a very minor behavioral response-5,9,7. In order to verify the in vivo heat response, WT and VR1-/- mice were placed on hot plates and their response to intense heat (>50°C) was measured by time taken to withdraw their paws. WT mice withdrew their paws quickly, but VR1-/- mice took roughly ten seconds longer to do so5.
These results proved that VR1 mediates the responses to pain and heat stimuli in vivo. The more interesting result, was that in the paw licking and paw withdrawal tests, the VR1-/- mice showed residual behavioral responses in regard to the pain and heat stimuli which they were presented with. This drew my lab’s attention into thinking that there may be more than one channel that is responsible for mediating responses to pain and thermal stimuli.
New Heat Channel
In 1977 Dubner et al. proposed that multiple classes of TRP channels detect heat.4. Because the VR1-/- mice showed residual responses to high temperatures, our lab searched the GenBank to find other DNA sequences related to VR1. Most of the sequence tags viewed, encoded for mouse and human orthologs of the same protein, which we named vanilloid-receptor-like protein (VRL-1)4. When mammalian cells transfected with VRL-1 were treated with heat (43°C), no current was evoked. When temperatures were increased higher (>53°C) an intense current was seen. Additionally, mammalian transfected VRL-1 cells treated with capsaicin did not show any response6. These results further support the idea that rather than having channels that deal in all ranges of thermal recognition, there are multiple channels that are involved in mediating the responses of a wide range of thermal stimuli.
New Developments in Pain
We previously established that along with residual responses to heat in mice lacking the VR1 receptor, the deficient mice also displayed residual responses to pain stimuli. This suggests that there may be additional channels that are involved in mediating the pain pathway. In 2004, Bandell et al. conducted experiments that determined that TRPA1, a family of TRP receptor channels, can be activated by using isothiocyanate compounds. These are molecules found within products like mustard oil and wasabi10, 11, 12. Like capsaicin, wasabi and mustard oil elicit inflammatory pain when consumed. Our lab demonstrated that the TRPA1 channel mediates different responses in comparison to VR1 in the pain pathway.
When cultured neurons were treated with mustard oil, roughly thirty percent of neurons responded in TRPA+/+ neurons, while TRPA-/- neurons did not respond11. This implies that the TRPA1 channel is the sole target through which isothiocyanate compounds use. Additionally, in vivo mouse models suggested thatTRPA1 is a mediator in the pain pathway. When WT mice had mustard oil injected into their paws, they withdrew their paws faster than mice lacking the TRPA1 receptor11.
In 2005, our lab conducted a different experiment using allicin, which gives garlic its spicy aroma. When cultured DRG neurons expressing VR1+/+ and VR1-/- were treated with allicin, there was no observed difference in the percentage of neurons that responded13. Additionally, when TRPA1+/+ channels were treated with allicin, they evoked a strong current, while TRPA1 deficient channels did not evoke any current13. These results further confirm that TRPA1 is involved in a different signaling pathway than VR1.
TRPA1 and the Formalin Model
Next, we explored the formalin-induced pain model. The formalin model was created over thirty years ago in order to determine pain and to test analgesic drugs14. The formalin model is important because it allows for pain assessment to be monitored in animals that are free to move around14. Yet, despite the popularity of this test, the mechanism of how it works has remained unexplained since its development. The main ingredient in this test is formaldehyde. In 2006, Hinman et al. performed experiments using molecules that have similar structures to formaldehyde, in order to activate TRPA1 channels14.
Using human and rat DRG and TG, we demonstrated that TRPA1+/+ treated with the formalin solution evoked calcium currents. However, when the mouse and human TRPA1-/- were treated with formalin, there was no current evoked. This further suggests that the pain pathway uses different channels in order to mediate diverse classes of pain stimuli 11, 14.
Next, WT mice that have the homologous TRPA1 channel, were injected with the formalin solution. They then licked their paw as a response to the painful stimulus. When mice lacking the TRPA1 receptor were injected with the formalin solution, there was a minimal behavioral response observed14. Finally, when TRPA1 channels were treated with allyl isothiocyanate, the evoked currents were almost identical to those evoked by channel treatment with formalin solution14. Stemming from this result, our lab decided to use a known antagonist of allyl isothiocyanate, HC-030031, to block the activation of TRPA1 by formalin solution14, 15. This proved to be successful, and the response of the WT mice with the antagonist injection had an identical response to formalin as the TRPA1 deficient mice14. This result is important because it allows TRPA1 to be studied as a target for modern analgesics, since it blocks the symptoms that are normally accompanied with tissue damage in animals.
Conflicting Ideas
There is an emerging conflict about the specific function of the TRPA1 channel. Jacquemar et al. 1999, Story et al. 2003, Bandell et al. 2004 and Jordt et al. 2004 all propose that TRPA1 functions in the mediation of cold stimuli, but our lab does not agree with that statement16, 12. In our TRPA1 vs cold sensation experiment, we placed zebrafish larvae that expressed TRPA1+/+, TRPA1+/-, and TRPA1-/- in very hot (>37°C), very cold (<16°C), and ambient temperature (~25°C) for their species. All of the larvae exhibited the same behavioral response in each of the different tempered waters12. Although our lab demonstrated that TRPA1 does not mediate noxious heat or noxious cold, there is a compelling amount of evidence that says otherwise. For this reason, more experiments on this subject should be carried out in the future to pinpoint exactly how TRPA1 channels mediate this thermal stimulus.
Cold and CMR1
Throughout our lab’s research, a great deal of information had been uncovered about the channel mediated thermal pathway. However, very little is still known about how cold temperatures are mediated via channels17. There isn’t a mechanism that has described how the process works. However, calcium-imaging patch clamp studies of dissociated DRG neurons, indicated that cold temperatures (20°C) induce a current via influx of calcium through ionotropic channels17. Although, in 1999 Suto et al. suggested that menthol binds to calcium channels, thereby activating them directly, there has not been any pharmacological or biochemical evidence of this phenomenon17.
Once again, our first line of approach was to use expression cloning in identifying the channel responsible for binding menthol. It was observed that menthol and cold activates a calcium channel in TG and DRG neurons of rats17. After the channel was isolated, subsequent experiments took place using Xenopus oocytes transfected with this channel. Menthol, as well as cold temperatures (8-28°C), activated the channel, evoking strong currents. However, when the channel was treated with capsaicin, no current was evoked17,18. These results imply that this channel is specialized for cold temperatures. Also, the fact that this channel is unresponsive to capsaicin, adds to the evidence that thermal sensitivity uses many different channels as mediators of temperature sensation. Because this channel is responsive to menthol and cold temperatures, we designated this channel as cold-menthol receptor type 1 (CMR1)17. Next, we conducted database searches in order to find a homology between this channel and others like it. We found that these channels are members of the TRP family. More importantly, it was concluded that the CMR1 receptor has a human orthologue TRPM817.
TRPM8 in Cold Sensation
As previously concluded, TRPM8 is a human orthologue of the rat CMR1 calcium permeable receptor, which dilates in response to cold, menthol or icilin17, 18. Icilin is a synthetic cooling agent, which has a much higher potency than menthol, and thus elicits a much stronger cooling sensation. Because icilin is a synthetic compound, we wanted to understand whether it behaves like a natural cooling agent such as menthol. When mammalian cells transfected with TRPM8 were treated with menthol, a strong current was evoked, Yet, the same channel, when treated with icilin failed to generate a current unless there was a presence of calcium ions external to the membrane1, 19. This result indicated that menthol and icilin act on TRPM8 through mechanically distinct pathways.
In vitro results successfully mapped out the mechanistic pathway of how sensation to cold temperature is mediated by TRPM8. The next approach to understanding TRPM8 was to see how essential it is in detecting cold temperatures. In order to do this, we generated TRPM8 deficient mice, by deleting a region in the mouse genome that encodes for the amino acids 594-66118. We saw that TRPM8 deficient mice DRG showed a loss of sensitivity to cold and menthol in vitro19,18, 20, 21. This same response was seen when tested in vivo. Mice deficient in TRPM8 showed a great reduction of flinching in response to the evaporative cooling effect after acetone application18. Interestingly enough, when temperatures were lowered below 10°C on a thermal plate, TRP+/+ and TRPM8-/- mice did not differ in their latency to engage in licking, in order to ease the strong cold stimulus18. These results suggest that TRPM8 may not be the sole channel responsible in mediating the cold sensation pathway, because some neurons are still being activated in response to low temperatures (<10°C).
VR1 Potentiation
Throughout our lab’s research, we have uncovered many mechanisms of nociception pathways. One thing that many of these pathways have in common is that channels like VR1 and TRPA1 can be potentiated in response to specific cues, such as protons and bradykinin22, 9, 11 27. These molecules are important in heightening an animal’s sense of pain as a warning signal that something is wrong.
Two key players in potentiating of channels are bradykinin and nerve growth factor (NGF). Both are pro-algesic agents that activate a cascade of signaling events, including the phospholipase C (PLC) pathway, which sensitizes channels to external stimuli. Although, the function of these molecules is known, there is no account of the molecular mechanisms that occur before a channel becomes permeable to ions.
First, we wanted to understand how essential the relationship between bradykinin, NGF and the VR1 channel is. We injected the paws of mice with NGF that were either VR1+/+ or VR1-/-,,and then these mice were subjected to heating. VR1+/+ withdrew their paws much quicker than the VR1 deficient mice9. The same result was seen when NGF was injected into the paws9. This result demonstrated that VR1 is essential for bradykinin and NGF function. In 2006, we conducted another experiment, in which bradykinin was injected into cultured TG neurons of mice transfected with WT, TRPA1-/- or TRPV1-/- (VR1-/-) channels. We observed that only the WT neurons were (VR1-/-) channels. It was seen that only the WT neurons were potentiated by bradykinin11. This suggested that both VR1 and TRPA1 need to function in order for sensitization via bradykinin to occur. Next, we began to experiment with the PLC pathway, because it was common to both NGF and bradykinin receptor activation. However, we did not know whether PLC stimulation was an obligatory step in VR1 potentiation9, 25. Using a tyrosine kinase A (TrkA) mutant, which abolishes the recruitment of PLC-γ to the VR1 receptor complex, we saw that there was a significant reduction in VR1 potentiation, compared to the WT receptor. This comparison was in response to treatment with NGF in VR1 transfected oocytes9. Next, direct application of PLC to patches excised from VR1-expressing HEK cells, caused an enhanced response of VR1 dilation as well as a stronger current elicited by capsaicin9. Judging from the responses of VR1 to a decreased or increased level of PLC, this demonstrates that the activation of PLC is the most important factor in potentiating VR1 in response to NGF.
In 2001, Chuang et al. suggested that phosphatidylinositol-4,5-biphosphate(PIP2), a VR1 channel gater, detects sensory stimuli and activates TRPV1 channels via its hydrolysis by PLC25. Mutant VR1 channels were detected by increases or decreases in thresholds of pain or thermal stimuli25. It was seen that one mutant (TRPV1.IRK) increased channel permeability, even under low concentrations of capsaicin. Conversely, the other mutant (TRPV1.IRK218Q) slightly reduced channel permeability25.
Overall, combining the results from each experiment, a molecular mechanism of the signaling pathway for VR1 channel sensitization was unfolded. Each step is crucial in maintaining the proper permeability of the channels in response to different stimuli. Thanks to the efficacy of the VR1 sensitization pathway, organisms are able to respond to dangerous environmental stimuli before they have the chance to injure themselves.
Proton Potentiation
When a human gets an injury like a bruise or a scrape, it is natural to assume that the individual will feel an exaggerated pain response when touching the affected area. In 1977, Jacobus et al. determined that the level of protons increases, in response to tissue injury such as infection and inflammation2. The previous statements suggest that increased levels of protons cause a lowering of internal pH,
thereby potentiating pain receptor channels to become more sensitized to stimuli. In order to explore this in-depth, my lab used cultured mammalian cells, expressing the VR1 receptor as a model to explore how acidity affects channel sensitization. When VR1 was treated with temperatures below its threshold and a neutral pH, there was no current evoked. When the pH was made more acidic a current was evoked. This implies that the increased level of protons potentiated the channel towards the heat stimulus, even though it is below the normal threshold (37°C) 2, 22. When the same experiment was done in a dose dependent manner, VR1 evoked currents with increasing strength, linearly with the increasing temperature and decreasing pH2,22. Thus, these results confirm that injuries result in increased pain sensation due to the drop in pH.
VR1 and Vasodilation
Anandamide is a cannabinoid receptor agonist and a very powerful vasodilator, but its mechanism remains unclear6. Because anandamide is a strong vasodilator, we believed that vasodilation resulted in the release of CGRP, which are vasodilator neuropeptides6.When anandamide was present in the arteries of rats and guinea pigs, CGRPs were seen in high quantities along with relaxation and dilation of the rodent arteries6.
TRP Structure: Coiled Coils
Many mechanistic pathways have been developed to explain how different thermal and painful stimuli are mediated by channels, however, there is little known about TRP channel structure. Our lab demonstrated that coiled coil domains are particularly important in the in intra- and inter-subunit interactions, which are essential for proper channel folding and maturation24. In an oocyte, a TRPM8 mutant that lacked a C-terminal coiled coil was transfected and treated with menthol. Unlike WT TRPM8, there was no current evoked. This was due to a mutation that made the channel unstable for menthol binding24. This result implies that further research should be conducted on channel structure.
Knowing small details of how channels function, can help in understanding future treatments that can be done in order to develop healthier channels.

Protein Channel Evolution
During our research, my lab focused on many aspects of channel function in different animals. During some of the research experiments, our lab encountered some slight differences between channel-mediated activities in different organisms. We think these differences may be have arisen throughout time via evolutionary adaptations. When looking at rat VR1 (rVR1) channels compared with avian VR1 (cVR1), there are many functional similarities, but rVR1 and cVR1 are essentially different26. rVR1 has a 68% identity and 79% similarity to cVR1. Mammalian cells transfected with VR1 and chick cells transfected with cVR1 both evoke currents in acidic conditions and high temperatures (43°C). But when the cells are treated with capsaicin, cVR1 does not evoke a current, while rVR1 does24. These results make evolutionary sense, because birds may be favored as vectors in transporting the seeds of spicy peppers, while mammals get repelled from the pungency. In this way, the seeds from the peppers can be distributed over longer distances.
Even more interesting is the difference in thermal response between warm and cold-blooded animals. Obviously, warm-blooded animals maintain a constant body temperature year round of roughly 37°C. Cold blooded animals, like frogs, have a fluctuating internal body temperature that mimics environmental conditions. In this experiment, we observed the differences in the cold receptor TRPM8 between rats and frogs. In Xenopus oocytes transfected with TRPM8, thermal activation of the receptor doesn’t occur until temperatures reach below 12°C. On the other hand, rat and chick TRPM8 were activated at much higher temperatures of 25 and 30°C27. These results make sense, because the activation of TRPM8 in Xenopus corresponds to their lowering of body temperature, while rats and chicks do not have that ability27.
Conclusion
Nociception is part of our everyday lives. Without sensation, we would not be who we are today. Without the ability to touch, no one would survive in this world. You would not have the ability to feel when you are injured, know when you have a severe burn, or even realize that you are touching boiling water. Since the beginning of my research, I would have never guessed the intricate workings of protein channels and how multiple stimuli can affect channels in different ways. Capsaicin is like the Drosophila of the nociception world. Using this substance has paved the way in understanding heat, pain, vasodilation, and sensitization pathways that account for many different mechanisms. My contribution to the field opened up new doors for how proteins should be viewed. One protein does not take care of all the work. It is many proteins working within one system, sometimes many proteins working just to open up one channel. TRPM8, VR1, TRPA1, and CMR1 are just the beginning in understanding how pain and temperature are perceived. In the future, I would like to conduct more experiments, in order to find new channels that will be responsible for lower thresholds of noxious cold and higher thresholds for noxious heat. I think that studying the proteins that bacteria in hot springs possess, will also shine a light on new ideas.
Acknowledgements
I would like to thank Dr. Shubhik DebBurman for his patience and unending support throughout the writing of this review. I would also like to thank Pete Sullivan for his editorial comments and the BIO 346 class for being supportive like a family throughout the entire semester.
References
1 Caterina MJ, Schumacher MA, Tominaga M, Rosen TA, Levine JD, Julius D. The capsaicin receptor: a heat-activated ion channel in the pain pathway. Nature. 1997 Oct 23;389(6653):816-24.
2 Basbaum, AI, D. M. Bautista, G. Scherrer, and D. Julius. “Cellular and molecular mechanisms of pain.” Cell 139.2 (2009): 267-84. Print.
3 Tominaga M, Caterina MJ, Malmberg AB, Rosen TA, Gilbert H, Skinner K, Raumann BE, Basbaum AI, Julius D. The cloned capsaicin receptor integrates multiple pain-producing stimuli. Neuron. 1998 Sep;21(3):531-43.
4 Caterina MJ, Rosen TA, Tominaga M, Brake AJ, Julius D. A capsaicin-receptor homologue with a high threshold for noxious heat. Nature. 1999 Apr 1;398(6726):436-41
5 Caterina MJ, Leffler A, Malmberg AB, Martin WJ, Trafton J, Petersen-Zeitz KR, Koltzenburg M, Basbaum AI, Julius D. Impaired nociception and pain sensation in mice lacking the capsaicin receptor. Science. 2000 Apr 14;288(5464):306-13.
6 Zygmunt PM, Petersson J, Andersson DA, Chuang H, Sorgard M, Di Marzo V, Julius D, Hogestatt ED. Vanilloid receptors on sensory nerves mediate the vasodilator action of anandamide. Nature. 1999 Jul 29;400(6743):452-7.
7 Siemens, J., S. Zhou, R. Piskorowski, T. Nikai, E. A. Lumpkin, A. I. Basbaum, D. King, and D. Julius. “Spider toxins activate the capsaicin receptor to produce inflammatory pain.” Nature 444.7116 (2006): 208-12. Print.
8 Bautista, D. M., Y. M. Sigal, A. D. Milstein, J. L. Garrison, J. A. Zorn, P. R. Tsuruda, R. A. Nicoli, and D. Julius. “Pungent agents from Szechuan peppers excite sensory neurons by inhibiting two-pore potassium channels.” Nature Neuroscience 11.7 (2008): 772-79. Print.
9 Chuang, H., Prescott, E.D., Kong, H., Shields, S., Jordt, S.E., Basbaum, A.I., Chao, M.V., and Julius, D. (2001) Bradykinin and nerve growth factor release the capsaicin receptor from PtdIns(4,5)P2-mediated inhibition. Nature 411: 957-962.
10 Hinman, A., H. H. Chuang, D. M. Bautista, and D. Julius. “TRP channel activation by reversible covalent modification.” Proceedings of the National Academy of Sciences of the United States of America 103.51 (2006): 19564-9568. Print.
11 Bautista, D. M., S. E. Jordi, T. Nikai, P. R. Tsuruda, A. J. Read, J. Poblete, E. N. Yamoah, A. I. Basbaum, and D. Julius. “TRPA1 mediates the inflammatory actions of environmental irritants and proalgesic agents.” Cell 124.6 (2006): 1269-282. Print.
12 Prober, D. A., S. Zimmerman, B. R. Myers, and B. M. McDermott. “Zebrafish TRPA1 channels are required for chemosensation but not for thermosensation or mechanosensory hair cell function.” Journal of Neuroscience 28.40 (2008): 10102-0110. Print.
13 Bautista, D. M., P. Movahed, A. Hinman, H. E. Axelsson, O. Sterner, E. D. Hogestatt, D. Julius, S. E. Jordt, and P. M. Zygmunt. “Pungent products from garlic activate the sensory ion channel TRPA1.” Proceedings of the National Academy of Sciences of the United States of America 102.34 (2005): 12248-2252. Print.
14 McNamara, C. R., J. Mandel-Brehm, D. M. Bautista, J. Siemens, K. L. Deranian, M. Zhao, N. J. Hayward, J. A. Chong, D. Julius, M. M. Moran, and C. M. Fanger. “TRPA1 mediates formalin-induced pain.” Proceedings of the National Academy of Sciences of the United States of America 104.33 (2007): 13525-3530. Print.
15 Trevisani, M., J. Siemens, S. Materazzi, D. M. Bautista, R. Nassini, B. Campi, N. Imamachi, E. Andre, R. Patacchini, G. S. Cottrell, R. Gatti, A. I. Basbaum, N. W. Bunnett, D. Julius, and P. Geppetti. “4-Hydroxynonenal, an endogenous aldehyde, causes pain and neurogenic inflammation through activation of the irritant receptor TRPA1.” Proceedings of the National Academy of Sciences of the United States of America 104.33 (2007): 13519-3524. Print.
16 Jordt, S. E., D. M. Bautista, H. H. Chuang, D. D. McKemy, P. M. Zygmunt, 16. E. D. Hogestatt, I. D. Meng, and D. Julius. “Mustard oils and cannabinoids excite sensory nerve fibres through the TRP channel ANKTM1.” Nature 427.6971 (2004): 260-65. Print.
17 McKemy, D. D., W. M. Neuhausser, and D. Julius. “Identification of a cold receptor reveals a general role for TRP channels in thermosensation.” Nature 41.6876 (2002): 52-58. Print.
18 Bautista, D. M., J. Siemens, J. M. Glazer, P. R. Tsuruda, A. I. Basbaum, C. L. Stucky, S. E. Jordt, and D. Julius. “The menthol receptor TRPM8 is the principal detector of environmental cold.” Nature 448.7150 (2007): 204-08. Print.
19 Chuang, H. H., W. M. Neuhausser, and D. Julius. “The super-cooling agent icilin reveals a mechanism of coincidence detection by a temperature-sensitive TRP channel.” Neuron 43.6 (2004): 859-69. Print.
20 Julius D, Basbaum AI. Molecular mechanisms of nociception. Nature. 2001 Sep 13;413(6852):203-10.
21 Jordt, S. E., D. D. McKemy, and D. Julius. “Lessons from peppers and peppermint: the molecular logic of thermosensation.” Current Opinion in Neurobiology 13.4 (2003): 487-92. Print.
22 Jordt SE, Tominaga M, Julius D. Acid potentiation of the capsaicin receptor determined by a key extracellular site. Proc Natl Acad Sci U S A. 2000 Jul 5;97(14):8134-9.
23 Bautista, D., and D. Julius. “Fire in the hole: pore dilation of the capsaicin receptor TRPV1.” Nature Neuroscience 11.5 (2008): 528-29. Print.
24 Tsuruda, P. R., D. Julius, and D. L. Minor. “Coiled coils direct assembly of a cold-activated TRP channel.” Neuron 51.2 (2006): 201-12. Print.
25 Prescott, E. D., and D. Julius. “A modular PIP2 binding site as a determinant of capsaicin receptor sensitivity.” Science 300.5623 (2003): 1284-288. Print.
26 Jordt, S. E., and D. Julius. “Molecular basis for species-specific sensitivity to “hot” chili peppers.” Cell 108.3 (2002): 421-30. Print.
27 Myers, B. R., D. Julius, and Y. M. Sigal. “Evolution of thermal response properties in a cold-activated TRP channel.” PLoS One 5th ser. 29.4 (2009). Print.
News Contact
[Role-played David Julius, Program in Biological Sciences, Department of Physiology, University of California, San Francisco, San Francisco CA 94158-2517]This author wrote the paper for Biology 346: Molecular Neuroscience taught by Dr. Shubhik DebBurman