The Intruding Ways of NF1, NF2, and Glioma Tumors
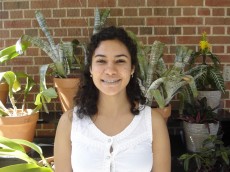
Sydni Cole, Daysi Vargas, Shabana Yusufishaq
Department of Biology
Lake Forest College
Lake Forest, Illinois 60045
Download PDF
Abstract
Cancer is among the top leading causes of death worldwide. Although more than one hundred types of cancers exist, those that afflict the nervous system are among the leading causes of cancer death in females in the United States. This review will focus on three major types of brain and nervous system cancers: gliomas and Neurofibromatosis types 1 and 2 (NF1, NF2). Glioma tumors result from mutations in various genes expressing for growth factors, including TGFβ, transcription factors including C/EBPβ and STAT3, gene products of the TROY and IDH1 gene, and cell cycle regulators such as p53. The epidermal growth factor receptor (EGFR) and the type III β3 isoform of neuregulins induce NF1 tumor formation. NF1 tumor formation occurs with a loss of heterozygosity (LOH) in Schwann cells and in the presence of mast cells heterozygous for NF1. NF2 inactivation via merlin calpain-dependent proteolysis, point mutations and ezrin overexpression can result in schwannomas and meningiomas formation. NF2 tumor formation and growth can be prevented by merlin activation, which can prevent cyclin D1 expression and mTORC1 activation. Further studying the causes of these types of tumors will allow for better treatment options to be developed.
Introduction
An astounding 1 in 4 individuals is diagnosed with cancer in the United States each year (Jemal et al., 2010). Cancer covers a spectrum of diseases characterized by the development of tumors, which form after uncontrollable cell proliferation due to a malfunction in the cell cycle (Fig. 1). The cause of cancer is not fully understood; however, cancer has strong genetic links that can act alone or can be triggered by environmental factors.
Some of the most common cancers are breast, prostate, colon, and lung cancer, all of which are the leading causes of cancer death. Although these cancers are extensively studied, 2% of cancer deaths in females are caused by brain and nervous system cancers (Jemal et al., 2010).
The three most common types of brain and nervous system tumors are gliomas linked to glial cell malfunction and Neurofibromatosis 1 and 2 linked to genetic alterations in the NF1 and NF2 genes.
Gliomas represent 32% of all brain tumors (http://www.abta.org). They originate from glial cells that provide nourishment to neurons, and can include astrocytes, oligodendrocytes, and ependymal cells. Gliomas occur in both primary and secondary forms, with primary, the most common form of brain tumors, originating in the brain (Gladson et al., 2010) and secondary originating from any other part of the body (Gladson et al., 2010; Huse et al., 2010). Glioblastoma multiforme (GBM) tumors are primary and are the most severe form of brain tumors (Huse et al., 2010). Some symptoms of gliomas, including glioblastoma, are nausea, vomiting, headaches, and seizures (Gladson et al., 2010). Individuals with glioblastoma may only live up to 18 months, while other glioma patients have survivorship up to 8 years (Gladson et al., 2010).
In contrast to glioma formation, neurofibroma tumors, which can be benign or malignant, form in the peripheral nervous system (PNS) due to mutations in the NF1 gene, which prevent tumor suppression (Zhu et al., 2002). Mutations in the NF1 gene are characteristic of Neurofibromatosis type 1 (NF1), which affects 1 in 3,500 people worldwide. Other symptoms of patients include café-au-lait macules, which are brown areas on the skin, learning disabilities, and cardiovascular problems (Rubin et al., 2005).
Similar to NF1, Neurofibromatosis type 2 (NF2) is an autosomal dominantly inherited disorder (Kimura et al., 1998; Ruttledge et al., 1994). NF2 is characterized by the formation of tumors such as schwannomas, ependyomas and meningiomas in the central nervous system (CNS) (Kimura et al., 2000, Ruttledge et al., 1994, Gareth and Evans, 2009). NF2 is often triggered by loss of heterozygosity (LOH), which is a loss of function of both alleles, and inactivation mutations in the NF2 gene (Chang et al., 2009). Neurofibromatosis type 2 affects 1 out of 60,000 individuals (Gareth and Evans, 2009). The development of NF2 tumors usually causes symptoms that are related to nerve damage such as hearing loss, vertigo, nausea, and vomiting (Gareth and Evans, 2009).
Gliomas: Understanding predicts hope
When studies on gliomas are performed, often scientists will resort to studying the mechanisms of malignant gliomas, usually those of glioblastoma, the most severe form of gliomas, and tumors originating from astrocytes, representing 54% of gliomas (Gladson et al., 2010; http://www.abta.org). The studies reviewed here focus on glioblastoma. These tumors quickly leave patients in a detrimental state because the tumors not only metastasize, but also become angiogenic, eventually leading to brain function loss. Studies on glioblastoma will contribute to the understanding of gliomas as a whole, allowing for treatments. The analysis that follows provides some insight on how the tumor cells evolve the ability to not only proliferate, but to become angiogenic.
Proliferation culprit: TROY gene
To understand the mechanisms of tumor invasion, genes related to the proliferation of gliomas were discovered, one of which is TROY (Paulino, et. al., 2010). TROY is expressed largely during embryogenesis, but is well regulated during adulthood (Paulino, et al., 2010). TROY expresses for a cell surface receptor for cells that are migrating or proliferating (Paulino, et al., 2010). Increased levels of the TROY mRNA (Fig. 2) result in a direct linear relationship in severity of tumorigenesis and an inverse relationship with patient survivability (Paulino, et al., 2010). This relationship is fitting considering tumor cells rely on each other’s cell surface receptors for migratory direction. As a result of TROY’s involvement in proliferation and migration it was not surprising to see increased levels of TROY expression in glioblastoma cells, both in vitro and in humans (Paulino, et al., 2010). However, it was found that TROY acts through a cell-signaling pathway and requires the activity of Pyk2 and Rac1 to be functional—allowing for the proliferation of tumors. Therefore, inhibiting one of these cell-signaling components may very well become a potential means of cancer treatment.
Figure 1. Tumor Formation and Cell Division. a) In a healthy cell, mitosis follows a normal pathway, resulting in two daughter cells. b) Factors, including gene mutations, cell signaling abnormalities, carcinogens, and protein malfunctions, might contibute to cell division malfuction, uncontrollable cell division, and the development of tumors.
Another gene culprit, angiogenesis: IDH1
Most primary brain cancers have an IDH1 gene mutation (Dang et al., 2009). The IDH1 wildtype gene expresses for an enzyme that converts isocitrate to α-ketoglutarate, whereas the mutant converts α-ketoglutarate to 2-hydroxglutarate (2HG), causing havoc to the Kreb’s cycle (Fig. 2). IDH1 mutations in glioma tumors have been confirmed and an increase in 2HG levels in these cells was seen (Dang et al., 2009). Malignant glioma patients have an 80% chance of an IDH1 mutation (Dang et al., 2009). Interestingly, those individuals who have the disorder 2-hydroxylutaric aciduria also have elevated levels of 2HG and are more likely to develop brain tumors (Dang et. al, 2009). It is thought that 2HG’s presence increases radical oxidative species and therefore decreases mitochondrial function, lowering oxygen levels. Lowered oxygen levels can lead to increased blood vessel formation, a key characteristic of gliomablastoma. Perhaps a high level of 2HG plays a large role in angiogenesis, the formation of blood vessels. The relationship between 2HG and the formation of tumors may provide a means of predicting risks to tumor formation at earlier stages of tumorigenesis.
Unwelcomed guests C/EBPß and STAT: More ways for angiogenesis
It appears that gliomas result from the malfunction of cellular systems that normally act to keep cells healthy. C/EBPβ and STAT3 act to activate some parts of the immune response including inflammation (Carro et al, 2010). However, these proteins are not expressed everywhere in the body; one place where these transcription factors do not get expressed in high quantities is glial cells (Carro et al, 2010). Interestingly, in gliomas these transcription factors are found (Carro et al, 2010) (Fig. 2). Perhaps the transcriptional factors’ normal role of inducing an inflammatory response contributes to tumors’ ability to invade their surroundings. Overall, the presence of the two transcription factors leads to a mesenchymal phenotype, but the inhibition of both transcription factors reduces invasiveness and angiogenesis, predicting a potential treatment option (Carro et al, 2010).
TGFβ: more than a growth spurt
It is also thought that TGF-β plays a direct role in providing gliomas the ability to invade their surroundings (Wesolowska, et al., 2008). Glial cells are normally cells that provide neurons and other nervous system related cells maintenance and growth, providing reason as to why TGF-β has been found in gliomas (Fig. 2). In healthy cells there are mechanisms for regulating the growth factors through means of inhibition, but this function seems to become impaired during tumorigenesis (Wesolowska, et al., 2008). These growth factors allow tumor cells to go through angiogenesis where they invade their environment and anchor themselves through the use of blood vessels as mentioned earlier (Behzadian et al., 1998). It was found that TGF-β was the source of such invasion as invasions were absent when TGF-β was inhibited (Wesolowska, et al., 2008). Unfortunately, such inhibition of the growth factor resulted in an immune system backlash indicating the importance of some TGF-β availability in a cell (Wesolowska, et al., 2008). Instead, simply inhibiting a receptor in the cell signaling pathway of this growth factor, TβIIR, resulted in similar detriment to the invasion of the tumor cells, but did not cause a negative response from the body. Interestingly, growth factors increase in other types of cancers too (Wesolowska, et al., 2008). A potential treatment by means of inhibiting TβIIR may contribute to increased survivorship in cancer patients.
Some hope: TMZ drug activates self-defense
Patients with glioma tumors can resort to treatment, such as surgery, radiotherapy, or drugs (Ross et al., 2007). A recent study tested the viability of an alkylating drug (which prevents DNA replication in tumor cells), temozolomide (TMZ) (Ross et al., 2007). The purpose of the experiment was to determine how TMZ leads to the death of cells. The use of the drug first pinpointed a lesion O6 methylguanine (MeG) so called as a result of a nucleotide alteration due the use of the drug (Ross et al., 2007). This lesion was then linked to glioma cells’ ability to go through apoptosis. However, the lesion alone did not induce apoptosis. The lesions were further aggravated when the cells replicated, forming double-strand breaks (Ross et al., 2007). This then triggered apoptosis once FAS, a receptor of p53, was activated (Ross et al., 2007). This further implies that FAS and p53 are also involved in carrying out apoptosis (Ross et al., 2007). The levels of apoptosis increased when p53 was not mutated indicating that when p53 is present it increases the extent of cell death (Ross et al., 2007). In other words, the same amount of the drug for either mutant p53 or wildtype resulted in greater apoptotic activity for the wildtype p53 gene. This is the case because mutant p53 does not result in direct cell death instead it targets the mitochondrial pathway.
NF1: the common tumor afflicter
Studies of Neurofibromatosis type 1 (NF1) typically are conducted about benign peripheral nerve sheath tumors, or neurofibromas, as said tumors are the most highly occurring tumors in NF1. These tumors contain a multitude of cells, such as axonal processes, Schwann cells, fibroblasts, perineurial cells, and mast cells (Zhu et al., 2002). However, how these cells interact in tumors and other factors that contribute to tumor formation was still unknown. Thus, recent studies have focused on understanding which cells require a loss of heterozygosity (LOH), which cells are most important in tumorigenesis, and different factors that contribute to the tumor formation of said cells.
The all-important Schwann cells
Since it was unknown which cells in neurofibromas require LOH for tumor formation, it was hypothesized that the LOH in Schwann cells is what leads to neurofibroma development (Zhu et al., 2002). Zhu et al (2002) found that conditional knockout mice, NF1-/- in Schwann cells and NF1+/- in all other cells, had tumors with 100% penetrance. Additionally, mice with NF1-/- Schwann cells and NF1+/+ in other cells had substantially less tumor formation than those with NF1-/- Schwann cells and NF1+/- in other cells (Zhu et al., 2002).
Scientists also wanted to determine which cell type in neural crest/Schwann cell lineage is the target for the NF1 mutation and how a loss of NF1 in cells leads to neurofibroma formation (Zheng et al., 2008). It was determined that nonmyelinating Schwann cells are seen in much higher numbers in the hyperplastic mutant nerves than non-mutants; this is seen as glial fibrillary acidic protein (GFAP) is positive in more cells in the mutants than in the non-mutants (Zheng et al., 2008). Additionally, abnormal Remak bundles were seen, with more axons present per pocket in the mutant nerves than in the control nerves (Zheng et. al., 2008).
The above data indicates that Schwann cell LOH is necessary to elicit tumor formation (Fig. 2), that nonmyelinating Schwann cells are indicative of neurofibromas, and that Remak bundles may potentially be a “cell of origin for plexiform neurofibroma” (Zheng et al., 2008).
Some tumor cells from bone marrow?
NF1-heterozygous mast cells, which are found in bone marrow, have been associated with neurofibroma formation when also present with NF1-/- Schwann cells (Yang et al., 2008). Additionally, the c-kit receptor activation is necessary for the release of mast cells from bone marrow (Yang et al., 2008). Since it has been found that NF1 LOH in Schwann cells is not sufficient for tumor formation, a study performed by Yang et al (2008) hypothesized that bone marrow derived cells that contain the c-kit receptor and are NF1+/- are involved in neurofibroma formation.
When NF1+/- bone marrow was present with LOH in Schwann cells, even if all other cells were NF1+/+, the mice had a much shorter survival rate and the tumors isolated from the mice exhibited neurofibroma pathology such as wavy Schwann cells, collagen deposits, structural abnormalities, and mast cell infiltration (Yang et al., 2008). Thus, bone marrow derived cells seem to be another type of cell that, when heterozygous for NF1 and in the presence of LOH in Schwann cells, induces tumorigenesis (Fig. 2).
EGFR: seen in yet another tumor type
In addition to studying Schwann cells and mast cells, researchers wanted to know more about the possibility of activated oncogenes leading to tumorigenesis. The epidermal growth factor receptor (EGFR)’s expression in Schwann cells was thought to be important to neurofibroma or malignant peripheral nerve sheath tumor (MPNST) development, in addition to the possibility that a decrease of EGFR could reduce tumor growth (Ling et al., 2005).
Some mice that overexpressed human EGFR (hEGFR) in Schwann cells developed triton tumors (an example of an MPNST) and neurofibromas; these individuals had hEGFR enriched in the tumors (Ling et al., 2005). Additionally, the mice exhibited dysfunctional Schwann cells, accumulated collagen, and high numbers of disrupted small axonal fiber bundles, indicating neurofibroma pathogenesis (Ling et al., 2005). It was also was determined that lower amounts of EGFR activity delayed tumor formation (Ling et al., 2005).
It is not known if EGFR is present in high amounts in all neurofibromas or just in the transgenic mice above; however, lowering amounts of EGFR could be a therapeutic target for NF1.
β3 isoform: another tumor inducing factor
Neuregulins are proteins that typically bind to and activate ErbB receptor tyrosine kinases and cause cell-cell signaling. Type III isoforms of neuregulins are the only isoform to control PNS myelination and are involved in the formation of Remak bundles, which are associated with NF1 (Sanchez et al., 2009). As the type III β1a isoform has been associated with myelination, scientists wanted to study if the type III β3 isoform also had a role in myelination and potentially neurofibroma formation (Sanchez et al., 2009).
There was a large increase in numbers of nonmyelinating Schwann cells (nmSC) over time in transgenic mice overexpressing the type III β3 isoform (Sanchez et al., 2009). This was seen as ErbB3 was upregulated, suggesting an expanded Schwann cell population, and GFAP, a marker for nmSC, was also upregulated (Sanchez et al., 2009). Additionally, the number of axons per Schwann cell pocket in Remak bundles was increased in transgenic mice compared to wild type (Sanchez et al., 2009). There also was an increase in collagen in the transgenic mice compared to wild-type mice (Sanchez et al., 2009). These findings indicate tumorigenesis in the transgenic mice, thus denoting that the type III β3 isoform of neuregulins induces tumorigenesis.
Unfolding NF2’s Role in Cancer
Although NF2 is induced by LOH and inactivation mutations (Chang et al., 2009), targeting of merlin, the protein encoded by the NF2 gene, a tumor suppressor, can also contribute to NF2 inactivation and tumorigenesis (Kimura et al., 1998). Expression of merlin can arrest the cell cycle and prevent tumor growth (Xiao et al., 2005; James et al., 2009) (Fig. 2). Although NF2 has been extensively studied, there is still much to be learned regarding NF2 inactivation and merlin’s role in cancer.
NF2 inactivation: the good news for meningiomas and schwannomas
It has been reported that mutations and LOH cause NF2 inactivation in 40 – 60% of sporadic meningiomas (Chang et al., 2009). Recent studies conducted using single strand conformation (SSC) and direct sequencing analysis found 24 inactivating mutations in the NF2 gene located on chromosome 22 (Ruttledge et al., 1994) (Fig. 2). Deletion mutations, splice-site alterations, and nonsense mutations are among some of the NF2 inactivating mutations found in NF2 tumors (Ruttledge et al., 1994). These mutations lead to protein inactivation and truncation (Ruttledge et al., 1994) (Fig. 2), which might be the actual cause leading to tumorigenesis.
Are mutations the only contributors to NF2 inactivation?
Recently it was discovered that mutations in NF2 are not the only factors that can contribute to NF2 inhibition. Given that not all NF2 tumors express mutant NF2, it was important to test if there were other contributors to NF2 inhibition. In fact, post-translational regulation is another way to prevent NF2 encoded protein, merlin, from inhibiting tumorigenesis (Kimura et al., 1998). Calpain-mediated proteolysis of merlin is found in gliomas. Merlin digestion by calpain was seen in vitro and in vivo (Kimura et al., 1998). Given that calpain needs calcium to operate, researchers studied the effect that high amounts of calcium could have on merlin. The results showed an increase of merlin proteolysis by calpain as calcium concentrations increased (Kimura et al., 1998). In a test to determine whether merlin proteolysis contributed to NF2 tumors, researchers expressed merlin and calpain in tumors that did not have NF2 mutations (Kimura et al., 1998). The results indicated that the termination of merlin translation was premature in some of these NF2 mutant depleted tumors (Kimura et al., 1998). This study provided convincing evidence suggesting that calpain proteolysis could in fact be involved in NF2 tumor development. Nevertheless, it is possible that future therapies might involve channel-calcium blockage to inhibit calpain activity and suppress tumor growth (Kimura et al 1998).
Other current studies found evidence of ezrin’s implication in NF2 tumors. Ezrin, in comparison to NF2, has an oncogenetic activity that can enhance cell proliferation (Morales et al., 2010). Since NF2 is a tumor suppressor gene, it would be expected to observe less tumor growth when NF2 is active. In GBM cells, low expression of NF2 resulted in cell growth, which indicates NF2 is in fact a tumor suppressor gene (Morales et al 2010). When studying NF2 and ezrin interaction, a negative correlation was found between the two subjects. Overexpression of NF2 decreased ezrin . Interestingly, ezrin down-regulation did not prove to be the contributing factor to NF2 tumor suppressing activity (Morales et al 2010). However, ezrin overexpression caused cell proliferation particularly in cells expressing NF2 (Morales et al., 2010). Another finding provided evidence that ezrin overexpression in cells that contain NF2 cause Rac1 levels to increase (Morales et al., 2010). Since NF2 tumor suppressing activity might be induced via lowering high levels of Rac1, the overexpression of ezrin that leads to high level of Rac1 might override NF2 tumor suppression activity (Morales et al., 2010). Although these results offer new ways to target NF2 tumors, further research is needed to identify other factors involved in NF2 inactivation.
Merlin the key in tumor suppression
Previous studies have suggested that merlin carries out NF2’s tumor suppressor activity by preventing cell proliferation (Tikko et al., 1994; Lutchman et al., 1995; Xiao et al., 2005). However, the exact mechanism by which merlin prevents cell proliferation still needs to be better understood. A study done using adenovirus-mediated expression of merlin revealed that merlin can arrest the cell cycle and inhibit cell proliferation by down-regulating cyclin D1 . Cell cycle progression was arrested at G1 phase (Fig. 2), and DNA synthesis was reduced in cells that expressed AdNF2 or NF2 A518 (Xiao et al., 2005). This was possible because cells expressing AdNF2 or NF2 A518 reduced cyclin D1 . Interestingly, NF2’s effect on cyclin D1 activity is accomplished by inhibiting cyclin D1’s promoter (Xiao et al., 2005). This study provided evidence suggesting that down-regulation of cyclin D1 could be one of the main targets of merlin that can prevent tumor growth.
Another pathway by which merlin prevents cell growth is by negatively interacting with mTOR Complex 1 (mTORC1) (James et al., 2009). Cells that have a merlin deficiency express high levels of phosphorylation of S6 and mTORC1 activity, which resulted in an increase in tumor cell size (James et al., 2009). However, when WT NF2 was re-expressed in NF2 deficient tumor cells, S6 phosphorylation decreased (James et al., 2009). Intriguingly, rapamycin, an mTORC1 inhibitor, is also effective at blocking cell growth in merlin deficient meningiomas (James et al., 2009). These results provide strong evidence of NF2’s merlin involvement in tumor cell growth prevention. In addition, the discovery of rapamycin as a cell growth inhibitor via regulation of mTORC1 could be a possible new therapeutic target to prevent tumor cells growth (James et al., 2009).
Future Advances in brain and nervous system cancer research
There are a number of protein malfunctions that can potentially lead to gliomas. Currently, much of the work being conducted to understand gliomas tends to be independent of one another. Perhaps new research can focus on connecting what it is we know about glioma formation mechanisms, which will allow us to see how they are interrelated. For instance, it can be further examined if IDH1 mutations and TROY mutations are present at the same time or if C/EBPβ and STAT and TGFβ are dependent on one another to carryout angiogenesis.
Studies of NF1 have recently shown that nonmyelinating Schwann cells’ LOH is vital in tumor formation, as is heterozygosity in mast cells. Additionally, factors such as EGFR and type III β3 isoform of neuregulins play parts in tumor formation as well. However, the pathway for which other tumor constituents, such as fibroblasts and collagen, find their way into neurofibromas is yet unknown, as are the specific roles of these tumor components. Thus, this could be further studied. Also, a depletion of EGFR has been shown to reduce tumorigenesis, so this interaction should be further studied in the hopes of finding a treatment for neurofibromas. And lastly, the type III β3 isoform of neuregulins needs to be further studied, as the research done recently is just preliminary and could lead to a greater understanding of NF1.
Current research in NF2 has provided insight into some of the mechanisms involved in NF2 and merlin’s tumor suppression activity. For instance these studies showed that tumor cell growth inhibition and cell cycle progression was prevented by merlin via mTORC1 negative regulation and cyclin D1 downregulation. However, it is important to investigate other mechanisms that contribute or affect NF2 tumor suppressor activity. In addition, these studies mentioned the potential calcium channel blockage and rapamycin as a potential tumor treatment.
Overall, in the case of each of these types of tumor formation it is important to eventually implement the potential treatments in human studies to determine the effectiveness of possible therapies.
Figure 2. The Genetic Pathway of Nervous System Tumors: This figure depicts the way that glioma, NF1, and NF2 tumors develop. Gliomas form due to a malfunctioning protein, protein excess, presence of growth factors, or transcription factors. Neurofibromas (in NF1) develop when Schwann cells lose heterozygosity of NF1 and all other tumor cells (and especially mast cells) are heterozygous for NF1. Inactivation mutations in NF2 and merlin malfunction cannot arrest the cell cycle in tumor cells and contribute to tumor cell proliferation.
Conclusion
Brain and nervous system cancer includes gliomas, and neurofibromatosis types 1 and 2. Glioblastomas are the worst type of gliomas due to their ability to proliferate and invade other tissues. Defects in the NF1 and NF2 genes can result in loss of function of proteins that can enhance tumorigenesis. Recent studies showed that these three types of brain and nervous system tumors are not only caused by mutations in genes but other factors as well. Links between these three tumors have also been noted and further studies will help develop a better understanding of their interconnection. These findings are relevant to the field of cancer given that they can provide the answers to the development of new therapies.
Note: Eukaryon is published by students at Lake Forest College, who are solely responsible for its content. The views expressed in Eukaryon do not necessarily reflect those of the College. Articles published within Eukaryon should not be cited in bibliographies. Material contained herein should be treated as personal communication and should be cited as such only with the consent of the author.
Acknowledgements
We would like to thank Dr. DebBurman for his guidance in completing this paper. We would also like to thank Keith Solvang for his support. And, finally we would like to thank Lake Forest College and the school’s Biology Department.
References
Behzadian, M. A., Wang. X., Shabraway, M., Caldwell, B. R. (1998).
Effects of Hypoxia on glial expression of angiogensis-regulating factors VEGF and TGF-β. Glia, 24, 216-255.
Carro, S. M., Lim, K. W., Alvrez, J. M., Bollo J. R., Zhao, X., Snyder,
Y. E. (2010). The transcriptional network for mesenchymal transformation of brain tumors. Nature, 463, 318-325. doi: 10.1038/nature08712.
Chang, Z., Guo, L., Ahronowitz, I., Stemmer-Rachamimov, A., MacColin, M., and Nunes, F. (2009). A role for the p53 pathway in
the pathology of meningiomas with NF2 loss. Journal of Neurooncology, 91, 265 – 270.
Dang, L. White, D., Gross, S., Bennett, D., Bittinger, A. M., Driggers, M. E. et al. (2009). Cancer associated IDH1 mutations produce 2-hydroxyglutarate. Nature, 462, 739-744. doi: 10.1038/nature8617.
Gareth, D., and Evans, R. (2009). Neurofibromatosis type 2 (NF2): A clinical and molecular review. Orphanet Journal of Rare Diseases, 4,
Gladson, L. C., Prayson, A. A., Liu, W. (2010). The pathology of glioma tumors. Annual Review Pathology, 5, 33-50.
Gomez-Sanchez, J.A., de Armentia, M.K., Lujan, R., Kessaris, N., Richardson, W.D., Cabedo, H. (2009). Sustained axon- glial signaling induces Schwann cell Hyperproliferation, remak bundle myelination, and tumorigenesis. Journal of Neuroscience, 29, 11304-11314.
Huse, T. J., Holland C. E. (2010). Targeting brain cancer: Advances in the molecular pathology of malignant glioma and medulloblastoma. Nature, 10, 319-331.
Ishikawa, K., Takenaga, K., Akimoto, M. Koshikawa, N., Ymaguchi, A. Imanishi, H., Nakada, K. (2008). ROS-genrating mitochondrial DNA mutations can regulate tumor cell metastasis. Science, 320, 661-664. doi: 10.1126/science.1156906.
James, M.F., Han, S., Polizzano, C., Plotkin, S.R., Manning, B.D., Stemmer-Rachamimov, A.O., et al. (2009). NF2/Merlin is a novel negative regulator of mTOR complex 1 and activation of mTORC1 is associated with meningioma and schwannoma growth. Molecular and Cellular Biology, 29, 4250-4261.
Jemal, A., Siegel, R., Xu, J., and Ward, E. (2010). Cancer Statistics 2010. Cancer J Clin 60, 277-300
Kimura, Y., Koga, H., Araki, N., Mugita, N., Fujita, N., Takeshima, H., et al. (1998). The involvement of calpain-dependent proteolysis of the tumor suppressor NF2 (merlin) in schwannomas and meningiomas. Nature, 4, 915 – 922.
Kimura, Y., Saya, H., and Nakao, M. (2000). Calpain-dependent proteolysis of NF2 protein: Involvement in schwannomas and meningiomas. Neuropathology, 20, 153-160.
Lutchman, M., and G. A. Rouleau. (1995). The Neurofibromatosis type 2 gene product, schwannomin, suppresses growth f NIH 3T3 cells. Cancer Research, 55, 2270-2274.
Ling, B.C., Wu, J., Miller, S.J., Monk, K.R., Shamekh, R., Rizvi, T.A., et al. (2005). Role for the epidermal growth factor receptor in neurofibromatosis-related peripheral nerve tumorigenesis. Cancer Cell, 7, 65-75.
Morales, F.C., Molina, J.R., Hayashi, Y., and Georgescu, M. (2010). Overexpression of ezrin inactivates NF2 tumor suppressor in glioblastoma. Neuro-Oncology, 12, 528– 539.
Paulino, M. V., Yang, Z., Kloss, J. Ennis, J. M., Armstrong, A. B., Loftus, C. J., Tran, L. N. (2010). TROY (TNFRSF19) is overexpressed in advanced glial tumors and promotes glioblastoma cell invasion via Pyk2-Rac1 signaling. Molecular Cancer Research, September 2010, OF1- OF10.
Roos, W. P., Naumann, S. C., Wick, W. Weller, M., Menck, C. F. M., Kaina, B. (2007). Oncogene, 26, 186-197.
Ruttledge, M.H., Sarrazin, J., Rangaratnam, S., Phela, C. M., Twist, E., Merel, P., et al. (1994). Evidence for the complete inactivation of the NF2 gene in the majority of sporadic meningiomas. Nature, 6, 180-183.
Tikko, A., Varga, M., Ramesh, V., Gusella, J., and Maruta H. (1994). An anti-Ras function of neurofibromatosis type 2 gene product (NF2/Merlin). J. Biol. Chem., 269, 23387-2339.
Wesolowska, A. Kwiatkowska, A., Slomnicki, L. Dembinski, M., Master, A. Sliwa, M. Franciszkiewicz, K. et al. (2008). Microglia derived TGF-β as an important regulator of glioblastoma invasion-an inhibition of TGF- β-dependent effects by shRNA against human TGF- β type II receptor. Oncogene, 27, 918-930.
Xiao, G., Gallagher, R., Shetler, J., Skele, K., Altomare, D.A., Pestell, R.G., et al. (2005). The NF2 tumor suppressor gene product, merlin, inhibits cell proliferation and cell cycle progression by repressing cyclin D1 expression. Molecular and Cellular Biology, 25, 2384-2394.
Yang, F., Ingram, D.A., Chen, S., Zhu, Y., Yuan, J., Li, X., et al. (2008). Nf1-dependent tumors require a microenvironment containing NF1+/- - and c-kit-dependent bone marrow. Cell, 135, 437-448.
Zheng, H., Chang, L., Patel, N., Yang, J., Lowe, L., Burns, D.K., et al. (2008). Induction of abnormal proliferation by nonmyelinating Schwann cells triggers neurofibroma formation. Cancer Cell, 13, 117-128.
Zhu, Y., Ghosh, P., Charnay, P., Burns, D.K., Parada, L.F. (2002). Neurofibromas in NF1: Schwann cell origin and role of tumor environment. Science, 296, 920-922.