C. elegans Exposed to Calorie Restriction and Resveratrol Concurrently have Statistically Similar Lifespans to the Control
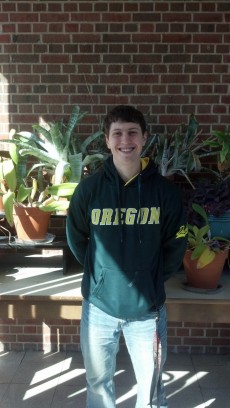
Tyler Kaplan
Department of Biology
Lake Forest College
Lake Forest, Illinois 60045
Download PDF
Background
Aging has become an important topic in the field of molecular biology in recent years and has led to the discovery of different methods that have been shown to increase life span in model organisms, such as Drosophilla melanogaster, Sacchromyces Cerevisiae, and Caenorhabditis elegans (Haigis & Sinclair, 2011). Two of the most well-known methods to extend life involve the calorie restriction (CR) diet and the small chemical resveratrol. Resveratrol is a polyphenol found in higher concentrations in grape skins and peanuts because it aids in preventing fungal infections (Kalantari & Das, 2010). Resveratrol levels tend to increase in the presence of oxidative stressors, such as heavy metal ions and UV radiation, possibly explaining why resveratrol has pro-longevity properties (Marques, Markus, & Morris, 2009). Calorie restriction reduces dietary intake by 20-50%. Unlike resveratrol, CR promotes longevity through under-nutrition not chemical properties. CR has been shown to increase the life in S. cerevisiae, C. elegans, D. melanogaster, M. musculus, and a variety of vertebrate fish by as much as 50% (Haigis & Sinclair, 2011). The study of lifespan extension of the model organism C. elegans by CR and resveratrol provides many advantages over other organisms. The nematodes are useful because they are small, have short life cycles, but most importantly are cheap and easy to culture. Additionally, they are eukaryotic and their pathways that appear to regulate aging are present in humans as well. Therefore, research on C. elegans can potentially have human applications as well.
We will be testing the effects of both CR and resveratrol independently as well as jointly to determine the effects of each individually and then to discover if together they are able to amplify their senescent defense properties. It has been conditionally shown in a variety of experiments that both resveratrol and CR can increase lifespan, but it remains unknown how the combination of both will affect the longevity of C. elegans. We believe that the groups exposed to both resveratrol and CR will have the largest increase in lifespan relative to the control group being grown in normal NGM plates. While CR and resveratrol tend to delay the onset of senescence in organisms, each condition can affect different genes. Additionally, genes such as the clk and daf genes appear to affect parallel pathways (Hekimi, 2000). C. elegans daf-2 clk-1 double mutants live longer than either strain with only one of the mutations (Hekimi, 2000). Thus, clk and daf genes appear to have an additive affect when each mutation is tested together (Hekimi, 2000). Although resveratrol and CR appear to have similar pathways, we still believe that there will be an additive affect of both on longevity.
Resveratrol and longevity:
Resveratrol increases the health of organisms by altering transcription of genes critical for longevity in a variety of ways. Many of these genetic alterations allow for increased protection from oxidative stressors, such as ultraviolet radiation (Kalantari & Das, 2010). Glutathione (GSH) is a powerful antioxidant, and resveratrol has been shown to increase levels of GSH Reductase, an enzyme that reduces the oxidized form of GSH to its functional form (Kalantari & Das, 2010). Resveratrol can improve the health of a variety of tissues especially the heart and the brain, through its pro-antioxidant effects. In the heart, mice given resveratrol had lower rates of apoptosis, improved left ventricle function, and better blood vessel formation (Kalantari & Das, 2010). Additionally, mice recovering from a myocardial infarction had an increase in the transcription of genes for blood vessels and capillaries. Nitric oxide is a vasodilator that causes the relaxation of smooth muscle in blood vessels causing the blood vessels to dilate resulting in decreased blood pressure. Mice treated with resveratrol showed increase nitric oxide levels, which is most likely a reason for many of the cardiovascular benefits of resveratrol (Kalantari & Das, 2010). Human studies looked at the cardiovascular benefits from moderate red-wine consumption in humans, because red-wine contains resveratrol. These results showed that individuals displayed an increase in vasodilation genes such as nitric oxide and a decrease in genes that are related to vasoconstriction (Marques et al., 2009). These individuals also had lower cholesterol than those who did not consume moderate amounts of red-wine. Resveratrol has the unique ability to improve brain tissue
Table 1: Comparisons of the blood work of people on CR and western diets. (Stein et al., 2012)
health because it penetrates the blood-brain barrier (Marques et al., 2009). The blood-brain barrier consists of tight junctions that make brain capillaries less permeable so certain chemicals are unable to enter the brain (Marques et al., 2009). Resveratrol in the brain helps prevent the build-up of beta amyloid, which is a protein that is often found excessively in patients diagnosed with Alzheimer’s disease. Resveratrol prevents beta amyloid buildup by preventing the protein from bonding to receptors on the plasma membrane of brain tissue (Marques et al., 2009). Thus, resveratrol acts as a natural defense to Alzheimer’s disease by inhibiting beta amyloid formation.
Resveratrol can also prevent the detrimental effects of a high-calorie diet. Mice were given a high-calorie diet (HC), a standard diet (SD), and a high-calorie diet with resveratrol (HCR). The HCR group maintained its quality of life similarly to that of SD mice because motor function improved in HCR mice until it was essentially the same as SD mice (Baur et al., 2006). In HC mice, livers increased in size, weight, and had large lipid deposits that reduced cellular integrity. However, HCR mouse livers did not develop any of these problems indicating that resveratrol helps maintain organ health (Barger et al., 2008). The HC group showed early-onset type II diabetes, while the HCR group had glucose levels that were statistically similar to the SD group. The HCR group also had better insulin sensitivity than the both the SD and HC group (Baur et al., 2006). The metabolic pathways of the HCR group looked almost identical to the SD group, not the HC group. The HC group had 153 altered genes in its metabolic pathways in comparison to the SD group, but the HCR group did not contain 144 of the genetic alterations that the HC group contained (Baur et al., 2006). Resveratrol appears to reduce the detrimental health benefits that are induced by a high-calorie diet.
Calorie Restriction mechanisms of life span extension:
Calorie Restriction extends longevity through alterations in metabolic and hormonal pathways (Barger et al., 2008). The key to properly performing CR is making sure that the diet is one of undernutrition, not malnutrition (Stein et al., 2012). Undernutrition provides fewer calories than normally required for an organism, while malnutrition results from the lack of one or more essential nutrients. Thus, the diet must provide all nutrients while restricting caloric intake. The effects of CR were compared to that of a western diet (WD). A western diet had 2400 kilocalories, while a CR diet had 1700 kilocalories (See Table 1). In both groups, nutrient intake was higher than the recommended daily dosages of the Federal Department of Agriculture. The subjects had been doing CR for multiple years prior to this study being conducted (Stein et al., 2012). The CR subjects had lower weights, body fat, blood pressure, and cholesterol. However, HDL was higher in the CR subjects than the WD group. In addition, the CR group had lower heart rates during all times of the day (Stein et al., 2012). Thus, it appears that CR subjects were healthier based on their healthier vital statistics. CR enhances bodily defenses against stressors as well (Kailasam et al., 2011). Calorie Restriction reduces oxidative stress by increasing antioxidant levels in cells (See Figure 1). Manganese Superoxide Dismutase (Mn SOD) and catalase are powerful antioxidant enzymes that fight against oxidative damage accumulation (Kailasam et al., 2011). Mn SOD converts superoxide anions to hydrogen peroxide, a reactive oxygen species that is less harmful than superoxide. However, catalase then converts hydrogen peroxide into oxygen gas and water (Kailasam et al., 2011). CR in Bcrachinonus plicatillis increased mRNA transcription of Mn SOD and catalase when oxidative
Figure 1: Calorie Restriction increases the response to ROS formation through a variety of antioxidants (Oberdoerffer & Sinclair, 2007)
stressors increased in cells (Kailasam et al., 2011). This increase in antioxidants was also observed in mice on CR indicating that CR does not increase the presence of antioxidants, but improves an organism’s ability to avoid oxidative damage (Kailasam et al., 2011). Although CR improves the health of organisms, the effects of CR vary based on tissue type. The mechanisms that mediate the effects of CR vary based on cellular specialization. White adipose tissue and heart tissue were monitored for how the transcription of known genes that are affected by CR differ. CR altered the transcription of more genes in the adipose tissue than the heart tissue. CR had few transcriptional changes in the heart, which is counterintuitive due to the multitude of cardiovascular benefits from CR. The cardiovascular benefits may be primarily caused indirectly by transcriptional changes in different tissues. However, both cells showed consistently similar levels in mammalian target of rapamycin (mTOR). In both cell strains, mTOR levels were conserved longer, while normally mTOR levels decline along with senescence. It is believed that mTOR may be a regulator for PPARy, a regulator of lipogenesis, mitochondrial biogenesis, and insulin sensitivity (Linford et al., 2007). Although CR improves insulin sensitivity, fasting glucose levels in standard diet groups were statistically equivalent to CR glucose levels (Yong-Ru et al., 2010). The levels of mTOR are similar over time, and PPARy suppression looks almost identical to mTOR suppression. Although mTOR is important in longevity, it appears that PPARy is far more important and instead mTOR is merely the activator for the protein. Additionally, the IGF-1 signal transduction pathway regulates PPARy levels, and there is an inverse relationship between the two proteins (Linford et al., 2007). Since CR decreases the metabolic activity of the IGF-1
Figure 2: Sir2 homolog in C. elegans, Sir-2.1 is activated by CR
induction (Longo & Kennedy, 2006)
pathway, PPARy levels increase. Calorie restriction delays the onset of a variety of other senescent indicators as well. The effects of calorie restriction delay the development of sarcopenia. Sarcopenia is the natural senescent based loss of muscle, and is typically seen in organisms (Marzetti, Lees, Wohlgemuth, & Leeuwenburgh, 2009). As aging occurs, the size and number of muscle fibers, typically type II or fasttwitch muscle fibers, decreases. This is due to changes in the collagen in the extracellular matrix (ECM) between muscle cells, which leads to increased cell fragility. Sarcopenia results from the oxidative damage caused by the formation of reactive oxygen species (ROS) from decreased mitochondrial functions. The formation of ROS causes mtDNA mutations due to the proximity of the electron transport chain to mtDNA. This decrease in mitochondrial function increase apoptosis because the apoptotic signaling mechanisms in mitochondria motion cell death. Although mitochondrial decline occurs in all cells, it is most observable in muscle cells because they contain the highest concentrations of mitochondria. CR delays sarcopenia by reducing mtDNA deletions and mitochondrial abnormalities in the ECM in mice. CR may slow down ROS formation by reducing proton leakage through the mitochondrial membrane and by increasing antioxidant transcription. Additionally, CR can alter the structure of the fatty acids that make up the mitochondrial membrane so that they better resist lipid peroxidation to prevent increased proton permeability. CR mice had increased mitochondrial formation, fewer mtDNA mutations, and less muscular atrophy than the controls (Marzetti et al., 2009). Rhesus monkeys placed on a CR diet also showed delayed sarcopenia and type II muscular atrophy (McKiernan et al., 2012). CR improves oxidative damage formation by mediating a special group of survival proteins.
Sirtuins:
Sirtuins are a group of proteins that provide a variety of defenses against oxidative damage. Research supports the claim that sirtuins are activated by resveratrol and are mediated by calorie restriction (Haigis & Sinclair, 2011). There are seven mammalian sirtuins (SIRT 1-7) (Baur et al., 2006). Sirtuins are classified as NAD+ dependent histone-deacetylases (Haigis & Sinclair, 2011). As respiration rate decreases the ratio of NAD+ / NADH increases, which then increases Sirtuin activity. CR regulates sirtuin activity because CR increases the NAD+/ NADH ratio (Skinner & Su-Ju, 2010). These proteins regulate other proteins that are essential in metabolism, such as insulin and cellular secretions (Haigis & Sinclair, 2011). SIRT 1 and SIRT3 can be induced through physical exercise because exercise increases lactic acid fermentation. As a result, NAD+ is replenished more readily and sirtuin activity increases. SIRT1, 6, and 7 are found in the nucleus, while SIRT3, 4, and 5 are found in mitochondria (Haigis & Sinclair, 2011). SIRT2 is found most commonly in the cytoplasm (Haigis & Sinclair, 2011). The location of the sirtuins is usually based on the specialization of the cell and the rate of its metabolic activity (Haigis & Sinclair, 2011). SIRT1, which is the most studied sirtuin, is the homolog of the Sir2 gene in S. cerevisiae and Sir-2.1 in C. elegans (See Figure 2). Removing Sir2 increased the accumulation of extrachromosomal rDNA circle (ERC) formation, which buildsup as S. cerevisiae age (Haigis & Sinclair, 2011). An additional copy of Sir2 increased replication by 30% and allowed S. cerevisiae to better deal with oxidative stress (Haigis & Sinclair, 2011; Oberdoerffer & Sinclair, 2007). Additionally, Sir2 can better prevent daughter cells from receiving ERCs from their mother by controlling the chaperonin proteins that fold actin, which is required to remove oxidized molecules from daughter cells (Haigis & Sinclair, 2011; Skinner & Su-Ju, 2010). These functions decline because senescence causes Sir2 to relocate from the nucleolus, which allows for increased ERC formation and decreased genomic stability (Haigis & Sinclair, 2011). Like sirtuins, CR and resveratrol extend life by affecting many similar metabolic pathways.
Connections between calorie restriction and resveratrol:
Calorie restriction and resveratrol may extend life through alterations in many of the same pathways. Studies in mice demonstrated that mice given resveratrol had similar metabolic markers to CR mice (Barger et al., 2008). Some 1029 heart genes showed different rates of transcription as senescence occurred. CR mice had 90% less of these age related transcription alterations, while resveratrol mice had 92% less age related changes in transcription than the control groups (Barger et al., 2008). Some 747 of these genes were common in both resveratrol and CR, while 745 of the 747 genes transcriptional patterns were similar in both the CR and resveratrol groups. This indicates that resveratrol and CR can delay many changes from age and they both work through preventing similar transcriptional changes. These results showed that low doses of resveratrol partially mimics CR because of the similar genetic transcription alterations observed in both experimental groups (Barger et al., 2008). Many of these genes are most likely involved in the regulation of cellular respiration rates, such as glycolysis, oxidative phosphorylation, and the IGF-1 pathway. These pathways are thought to be altered by CR and resveratrol and may aid in increasing longevity (Baur et al., 2006). By slowing down the rate of respiration, the onset of senescent related diseases may be delayed. Many of the results of studies conducted on mice were observed in C. elegans as well.
Studies on C. elegans:
C. elegans demonstrates a longer life when given resveratrol and calorie restriction. However, in some of the studies conducted, resveratrol did not increase SIR-2.1 transcription, the homolog of SIRT1 in mammals, which many other studies have observed as the mechanism for life extension (Bass, Weinkove, Houthoofd, Gems, & Partridge, 2007). Some argue that resveratrol increases life expectancy because of an increase in drug detoxification defenses that prevent damage caused by toxins and other free-radical causing substances (Bass et al., 2007). Thus, it is possible that resveratrol works through a different mechanism than CR, which can increase the lifespan of C. elegans by up to 50% (Baur et al., 2006). These studies that contradict the belief that resveratrol is a sirtuin activator provides us with the idea that both CR and resveratrol may improve the lifespan of C. elegans more than either of the factors individually. Ideally, the resveratrol and CR groups will exhibit the longest life spans indicating that resveratrol works on a different pathway than CR.
Methodology:
The N2 worm strain, the wild-type worm strain that has a normal insulin-signaling pathway and no other mutations, was used for this experiment. The wild-type strain was used because environmental factors that contribute to aging were tested, thus no Caenorhabditis elegans mutants were necessary. Two environmental factors were tested: calorie restriction and the anti-oxidant resveratrol. Both variables were tested both independently of each other and jointly. This was done in order to see if using both known methods of slowing the onset of senescence further enhanced longevity. The longevity of each group was compared to the control group that was given a normal calorie diet and was not exposed to resveratrol. All Petri
Table 2: The amount of worms alive were converted into a percentage
to better compare the amount of worms alive because each
culture had varying numbers of worms.
Figure 3: The graph shows the percentage of worms alive at each
assessment, all worms were alive on day one, and were the same
dishes contained the chemical FUDR to prevent C. elegans from reproducing. This was crucial because if C. elegans reproduced, it would have been impossible to track the lifespans of each worm. All solutions were sterilized using a 0.22 micron filter cassette to ensure that no microbes infected the petri dishes. These microbes could introduce competition into the environment of C. elegans for resources. 100 micrograms of resveratrol were added to NGM plates. This was determined from the typical dose of resveratrol taken in tablet form in humans.
100 micrograms, which is a slightly excessive amount, were added because we knew not all of the resveratrol would be absorbed by C. elegans. The calorie-restriction group was studied in low-calorie plates, which contained no nutrients in the agar plate. There were two Petri dishes for each group in case one Petri dish yielded poor results. Data was collected three times per week and we counted the amount of worms remaining in each Petri dish.
Discussion:
We hypothesized that the experimental group exposed to both resveratrol and CR would promote the greatest longevity because of a possible additive effect induced because both individually lengthened life span. We believed that resveratrol and CR could work similarly to the clk and daf genes in C. elegans, where when used together lengthen the lifespan of C. elegans longer than each used individually (Hekimi, 2000). As we expected, the resveratrol group and the CR groups lived longer and our data was statistically significant in comparison to the NGM group, with p-values of 7.39 x 10 -6 and 0.0334 respectively (See Table 2). The divergence between these groups occurred within the first three days of the worm counts (See Figure 3). Additionally, the CR and resveratrol groups did not display data that were significant to each other (See Table 2), therefore supporting Barger’s data that resveratrol partially mimics the effects of CR (Barger et al., 2008). Thus, resveratrol and CR possibly act on the same metabolic pathways and both appear to be activators for sirtuins, which many believe is a protein vital for delayed senescence (Haigis & Sinclair, 2011).
These results did not surprise us because they have been widely tested and the results have been relatively consistent throughout many studies. However, we were surprised by the CR and resveratrol data because when compared with the NGM group, it yielded a p-value of .891, indicating the data was not statistically significant (See Table 2). Even the lines for the NGM and CR with resveratrol groups display highly similar trends (See Figure 3). This was especially apparent in the first three days, where similarities in mortality rate were apparent (See Figure 3). The data was remarkably similar to the results obtained by the NGM group indicating that resveratrol and CR essentially negated the benefits of each individually. Unfortunately, it is very difficult to corroborate these results because there have been no experiments where both resveratrol and CR have been tested simultaneously.
These results can possibly be explained through the results obtained by Dr. Ristow on C. elegans as well. His data led to the conclusion that CR reduces the net ROS production, but in the short-term increases ROS production (Schulz, 2007). Glucose is required for glycolysis to continue to run. However, CR reduces glucose levels slowing down glycolysis and thus glycolytic ATP production. In order to compensate for this decrease in ATP production, mitochondrial ATP production increases, which then causes more ROS to be produced (Schulz, 2007). Resveratrol and CR both lead to an upregulation of the AMPK homolog aak-2 in C. elegans, which then increases respiration, which in turn increase ROS production (Greer & Brunet, 2009; Schulz, 2007) (See Figure 4). There could be possibly an additive effect from both CR and resveratrol on the activity of aak-2. In response to this short-term increase in ROS, the body increases the long-term expression of antioxidants such as Mn SOD and catalase (Kailasam et al., 2011). Previous studies have shown that AMPK activation is a crucial protein affected by both CR and resveratrol. Since resveratrol and CR appear to work through similar pathways, we propose that resveratrol and CR, by means of aak-2 activation, increased ROS to a point where rather than a decrease in net ROS production, there was no net ROS production. Therefore, ROS production would appear similar to that of the control because of the additive effect of ROS production from resveratrol and CR. Due to our results, we now suggest that the CR and resveratrol condition did not extend lifespan in C. elegans because there was no net decrease in ROS production that typically occurs with each variable independently.
There were two sources of uncertainty that could have affected the results of our study. Fungi infected some of our plates, which could have affected worm mortality and maybe shortened life-span. The CR with resveratrol group appeared like the control group possibly because fungi increased mortality rates. However, the resveratrol and CR groups each improved C. elegans life-span which makes it less likely that fungi only affected the CR with resveratrol group. Due to the uniqueness of our data, I would be interested in repeating this experiment with
Table 2: Greater than .05 p-value indicates statistically insignificant data, thus the data could have been obtained by chance.
the CR and resveratrol group. The CR with resveratrol condition was unique to this experiment, thus without this condition being retested, the current data received has little validity. In order to further support our data, I would want to repeat this experiment with only the CR with resveratrol condition to hopefully replicate the results we received. I still remain skeptical of our results because resveratrol and CR work through many of the same metabolic pathways
Figure 4: Calorie restriction upregulates aak-2 in C. elegans, which increases mitochondrial respiration causing an increase in ROS production. This stimulates an increased response to oxidative stressors (mitohormesis), and a longer term increase in antioxidants. However, too much glucose has the opposite effect
which led me to believe that each independent condition would be more similar than the NGM group to the CR with resveratrol group. Additionally, I would like to study the metabolic pathways of both resveratrol independently and simultaneously with CR to determine differences in each of their effects. Although our data should be taken with skepticism, it appears it is possible to have too much of a good thing.
Conclusion:
Our data suggests that resveratrol and CR used concurrently mimic the control through activation of the AMPK homolog aak-2 more than each does individually through an additive effect. This activation of AMPK stimulates an increase in mitochondrial respiration, which causes ROS formation. Typically, CR and resveratrol independently lead to an increased anti-oxidant production to reduce the net amount of ROS, but used together this increased ROS formation leads to no net change in ROS formation in comparison to the control. Thus this condition’s life span seemed similar to that of the control because of similar oxidative damage build-up.
Acknowledgements:
We would like to thank Professor Smith for all of his support throughout the entire research process. He provided us with all of the materials, which included the resveratrol, agar plates, worms, and most importantly lab space and equipment. Additionally, he taught us how to properly count the worms and aided us in making tools to handle the worms.
References
Barger, J. L., Kayo, T., Vann, J. M., Arias, E. B., Wang, J., Hacker, T. A., … Prolla, T. A. (2008). A low dose of dietary resveratrol partially mimics caloric restriction and retards aging parameters in mice. PLoS ONE, 3, 1-10.
Bass, T. M., Weinkove, D., Houthoofd, K., Gems, D., & Partridge, L. (2007). Effects of resveratrol on lifespan in drosophila melanogaster and caenorhabditis elegans. Mechanisms of Ageing & Development, 128, 546-552.
Baur, J. A., Pearson, K. J., Price, N. L., Jamieson, H. A., Lerin, C., Kalra, A., … Sinclair, D. A. (2006). Resveratrol improves health and survival of mice on a high-calorie diet. Nature, 444, 337-342.
Greer, E. L., & Brunet, A. (2009). Different dietary restriction regimens extend lifespan by both independent and overlapping genetic pathways in c. Elegans. Aging Cell, 8, 113-127.
Haigis, M. C., & Sinclair, D. A. (2011). Sirtuins in aging and agerelated diseases. In E. J. Masoro & S. N. Austad (Eds.), Handbook of the biology of aging (7th ed.). (pp.243-274). San Diego, CA US: Elsevier Academic Press.
Hekimi, S. (2000). The molecular genetics of aging. New York:Springer-Verlag. Kailasam, M., Kaneko, G., Oo, A. K. S., Ozaki, Y.,Thirunavukkarasu, A. R., & Watabe, S. (2011). Effects of calorie restriction on the expression of manganese superoxide dismutase and catalase under oxidative stress conditions in the rotifer brachionus plicatilis. Fisheries Science, 77, 403-409.
Kalantari, H., & Das, D. K. (2010). Physiological effects of resveratrol. Biofactors, 36, 401-406. Linford, N. J., Beyer, R. P., Gollahon, K., Krajcik, R. A., Malloy, V. L., Demas, V., … Rabinovitch, P. S. (2007). Transcriptional response to aging and caloric restriction in heart and adipose tissue. Aging Cell, 6, 673-688.
Longo, V. D., & Kennedy, B. K. (2006). Sirtuins in aging and agerelated disease. Cell, 126, 257-268. Marques, F. Z., Markus, M. A., & Morris, B. J. (2009). Resveratrol: Cellular actions of a potent natural chemical that confers a diversity of health benefits. International Journal of Biochemistry & Cell Biology, 41, 2125-2128.
Marzetti, E., Lees, H. A., Wohlgemuth, S. E., & Leeuwenburgh, C. (2009). Sarcopenia of aging: Underlying cellular mechanisms and protection by calorie restriction. Biofactors, 35, 28-35.
McKiernan, S. H., Colman, R. J., Aiken, E., Evans, T. D., Beasley, T. M., Aiken, J. M., … Anderson, R. M. (2012). Cellular adaptation contributes to calorie restriction-induced preservation of skeletal muscle in aged rhesus monkeys. Experimental Gerontology, 47, 229-236.
Oberdoerffer, P., & Sinclair, D. A. (2007). The role of nuclear architecture in genomic instability and ageing. Nature Reviews Molecular Cell Biology, 8, 692-702.
Schulz, T. J., Zarse, K., Voigt, A., Urban, N.. Birringer, M., & Ristow, M. (2007). Glucose restriction extends caenorhabditis elegans life span by inducing mitochondrial respiration and increasing oxidative stress. Cell Metabolism, 6, 280-293.
Skinner, C., & Su-Ju, L. (2010). Effects of calorie restriction on life span of microorganisms. Applied Microbiology & Biotechnology, 88, 817-828.
Stein, P. K., Soare, A., Meyer, T. E., Cangemi, R., Holloszy, J. O., & Fontana, L. (2012). Caloric restriction may reverse age-related autonomic decline in humans. Aging Cell, 11, 644-650.
Yong-Ru, C., Shi-Rong, F., Yu-Cai, F., Xiao-Hui, Z., Ming-Yan, X., & Wen-Can, X. (2010). Calorie restriction on insulin resistance and expression of sirt1 and sirt4 in rats. Biochemistry & Cell Biology, 88, 715-722.